Aquaponics – Multitrophic Systems for Sustainable Food Production
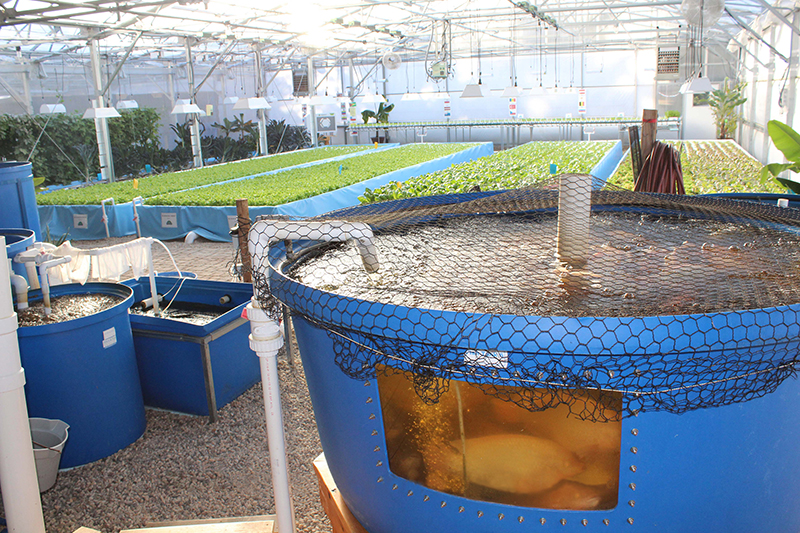
Aquaponic system. Photo: Nelson and Pade, Inc.
By Lee Rinehart, NCAT Agriculture Specialist
Abstract
Aquaponics is a bio-integrated system that links recirculating aquaculture with hydroponic vegetable, flower, and/or herb production. Advances by researchers and growers alike have turned aquaponics into a working model of sustainable food production. This publication introduces aquaponic systems, discusses economics and getting started, and includes an extensive list of resources that point the reader to print and online educational materials for further technical assistance.
Contents
Introduction
Aquaponics: Key Elements and Considerations
Economics and Business Planning for Aquaponics
Evaluating an Aquaponic Enterprise
Further Resources
References
Appendix I: Organic Aquaculture/Aquaponics
Appendix II: Aquafeeds and Their Alternatives
Introduction
Aquaponics, also known as the integration of hydroponics with aquaculture, is gaining increased attention as a bio-integrated, multitrophic food production system. This publication, originally written in 2000, has been updated to take into consideration the newest technologies, trends, and philosophies of aquaponic food production and system operation. It introduces aquaponics and provides extensive further resources. It does not attempt to describe production methods in comprehensive technical detail, but it does provide a summary of key elements and considerations. References to details on specific production techniques and methods, including manuals and technical information on specific systems, can be found in the Resources section.
Aquaponics serves as a model of ecological food production by following several basic principles:
- The waste products of one biological system serve as nutrients for a second biological system, facilitated by microbial activity.
- The integration of fish and plants results in a multitrophic polyculture that increases diversity and yields multiple products.
- Water is re-used through biological filtration and recirculation.
- Local food production provides access to healthy foods and enhances the local economy.
Microorganisms are the important link between aquatic animals and plants in an aquaponic system. Plants evolved in association with microorganisms, and having microbes in all parts of the aquaponic system builds an important connection between animals and plants that facilitates nutrient uptake and plant vigor. Nutrient-rich effluent from fish tanks is used to fertilize hydroponic production beds. This is good for the fish because plant roots and rhizobacteria remove nutrients from the water. These nutrients — generated from fish manure, algae, and decomposing fish feed — are contaminants that would otherwise build up to toxic levels in the fish tanks, but instead serve as liquid fertilizer to hydroponically grown plants. In turn, the hydroponic beds function as a biofilter — stripping off ammonia, nitrates, nitrites, and phosphorus — so the freshly cleansed water can then be recirculated back into the fish tanks. The nitrifying bacteria living in the gravel and in association with the plant roots play a critical role in nutrient cycling; without these microorganisms the whole system would stop functioning.
Table 1: Hydroponic and Aquaponic Comparison Chart. Adapted from Davidoc, 2016 | ||
Hydroponics | Aquaponics | |
Principles | Conventional | Biological |
Recirculation | Possible | Closed loop |
Source of nutrients | Synthetic fertilizers | Fish waste, nutrients released due to biological activity |
Beneficial microorganisms | Practically excluded | Essential part of the system |
Building multitrophic relationships in the system | To some extent | Yes |
Balance | One-component system | Balanced |
Self-regulation | No | Yes |
Creating an ecosystem | No | Yes |
Stability of a mature system | Not stable | Very stable |
Knowledge base | Plant physiology | Ecology, plants, fish, microorganisms |
Aquaponics harnesses a natural process and, unlike hydroponics, has the potential to be a sustainable, zero-waste food production system. “Soil is not the only biologically active and diverse medium,” notes Nick Savidov, an aquaponic researcher at Lethbridge College (2018). Like soil, a closed-loop aquaponic system is an ecosystem, and this is a change of paradigm for many hydroponic producers. Aquaponics is not just hydroponics plus aquaculture… it’s hydroponics plus aquaculture plus a diverse microbial community that facilitates nutrient cycling.
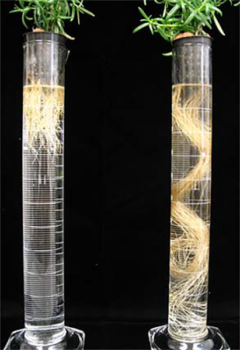
Rosemary roots grown hydroponically (left) and aquaponically (right). Photo: Nick Savidov
Just as adding fish to plants increases efficiencies, adding plants to land-based recirculation aquaculture has its own benefits. Recirculation aquaculture requires that waste water be treated to remove nutrients before discharge, usually into a local body of water. Recirculation aquaculture is expensive given the cost of fish feed, the cost to treat the water, and the costs associated with permitting and discharge. It also produces a waste stream that must be managed (NHPR, 2018). Adding plants to a recirculating aquaculture system introduces a natural biofilter that treats the water, which can then be recirculated back to the fish, making a closed system.
Though aquaponic systems capture nutrients from fish effluent and pass them through the plant component of the system for filtration, there are still some inefficiencies. The fish-waste solids left behind after biofiltration must still be handled. Currently, producers manage fish manure through field application, composting, and anaerobic digestion (Khiari et al., 2019). These practices, though they capture some nutrients, incur nutrient losses through greenhouse gas emissions and leaching. Recycling fish waste solids back into the aquaponic system would close the loop and reduce waste to practically zero.
One way to capture the nutrients in solid fish waste is being investigated by researchers at Lethbridge College in Alberta, Canada. Using aerobic bioreactors, they have developed a two-loop system for treatment of liquid and solid waste (Khiari et al., 2019). Since 2005, aerobic bioreactors have been used to break down and release mineral nutrients to loop back into the system, with remarkable results (Savidov, 2018). Whereas mineral supplementation is common in conventional aquaponics, the two-loop system doesn’t require this. The solid-waste nutrients that are captured in the process complement liquid-effluent nutrients, and this eliminates any waste in the system.
The technology associated with aquaponics is complex. It requires the ability to manage the production and marketing of two different agricultural products simultaneously. Historically, most attempts at integrated hydroponics and aquaculture had limited success. However, recent innovations have transformed aquaponics technology into a viable system of food production. Modern aquaponic systems can be highly successful, but they require intensive management and careful attention to business planning and marketing, as well as the use of technology for monitoring and system control.
Aquaponics: Key Elements and Considerations
A successful aquaponics enterprise requires special training, skills, and management. The following items point to key elements and considerations to help prospective growers evaluate the integration of hydroponics with aquaculture.
Greenhouse growers and farmers are taking note of aquaponics for several reasons:
- Hydroponic growers view fish-manured irrigation water as a source of organic fertilizer that enables plants to grow well.
- Fish farmers view hydroponics as a biofiltration method to facilitate intensive recirculating aquaculture.
- Greenhouse growers view aquaponics as a way to introduce organic hydroponic produce into the marketplace, because the only fertility input is fish feed, and all of the nutrients pass through a biological process.
- Food-producing greenhouses — yielding fish and produce from one production unit — are naturally appealing for niche marketing and green labeling.
- Aquaponics can enable the production of fresh vegetables and fish protein in arid regions and on water-limited farms, because it is a water re-use system.
- Aquaponics is a working model of sustainable food production, wherein plant and animal agriculture are integrated and recycling of nutrients and water filtration are linked.
- In addition to commercial applications, aquaponics has become a popular training aid on integrated bio-systems for vocational agriculture programs and high school biology classes.
Basic Aquaponic System Operation
As discussed, aquaponics combines two production components (fish and vegetables) to integrate feed and waste processes in a closed system. The basic components include a fish-rearing tank, where feed is consumed by fish and the nitrogen cycle begins. Nitrogen is taken up by the fish, metabolized into protein, and excreted into the water as waste. From there, nutrients in the water (feed and feces) are cycled to the settling tank, where solids are removed and can be added to compost. Next in line is a biofiltration tank, where ammonia from the waste nitrogen produced by the fish tank is converted to nitrite and then to nitrate by bacteria. Then, the plant-available nitrogen is pumped into grow beds, where nitrogen is taken up by plants and removed from the system in the form of produce. Waste water from the grow beds cycles to the sump, then is degassed (where oxygen is put in and carbon dioxide is taken out). Finally, the water is pumped back into the fish-rearing tank to begin the cycle once again.
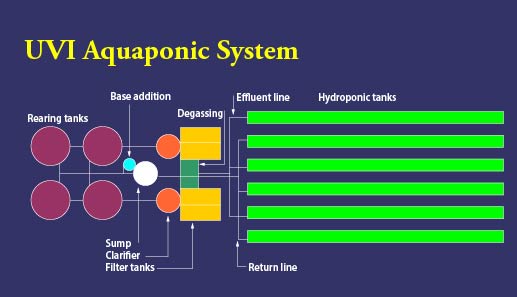
Table 2: UVI Diagram. Source: University of the Virgin Islands Aquaponics Program
Types of Aquaponic Systems
1. Nutrient film technique (NFT) allows the plant roots to absorb nutrients from a ½-inch film of water with high oxygen exposure. A small amount of water enters one end of a channel or gutter and flows by gravity to the other end, where it drains into a common collection area. Because of the high potential surface area, this method allows for greater plant production with less water.
Nutrient film technique. Photo: purehydroponics.com
2. Flood and drain uses of a substrate of pea gravel, perlite, or expanded clay as a plant growth medium. A biofilter is not necessary in this system, because bacteria colonize the internal structures and pipe in the system, providing essential nitrification of fish wastes for plant uptake. Water constantly flows through the system on a 20- to 30-minute cycle to provide periodic exposure of the plant roots to water and air. Flood and drain systems are particularly suited for heavy-fruiting plants such as peppers or tomatoes.
Flood and drain. Photo: Nelson and Pade, Inc.
3. Floating raft systems use a platform, usually polystyrene, to support the plants and allow the roots to access water underneath. As with flood and drain systems, a biofilter is usually not necessary. A large volume of water circulated to the roots creates temperature stability and better water quality.
Floating raft. Adapted from Burden and Pattillo, 2018. Photo: Texas AgriLife Extension
Source: Adapted from Burden and Pattillo, 2018
Hydroponics: Hydroponics is the production of plants in a soilless medium, whereby all of the nutrients supplied to the crop are dissolved in water. Liquid hydroponic systems employ the nutrient film technique (NFT), floating rafts, and noncirculating water culture. Aggregate hydroponic systems employ inert, organic, and mixed media contained in bag, trough, trench, pipe, or bench setups. Aggregate media used in these systems include perlite, vermiculite, gravel, sand, expanded clay, peat, and sawdust. Normally, hydroponic plants are fertigated (soluble fertilizers injected into irrigation water) on a periodic cycle to maintain moist roots and provide a constant supply of nutrients. These hydroponic nutrients are usually derived from synthetic commercial fertilizers, such as calcium nitrate, that are highly soluble in water. However, hydro-organics — based on soluble organic fertilizers such as fish hydrosylate — is an emerging practice. Hydroponic recipes are based on chemical formulations that deliver precise concentrations of mineral elements. The controlled delivery of nutrients, water, and environmental modifications under greenhouse conditions is a major reason why hydroponics is so successful.
Nutrients in Aquaculture Effluent: Greenhouse growers normally control the delivery of precise quantities of mineral elements to hydroponic plants. However, in aquaponics, nutrients are delivered via aquacultural effluent. Fish effluent contains sufficient levels of ammonia, nitrate, nitrite, phosphorus, potassium, and other secondary and micronutrients to produce hydroponic plants. Naturally, some plant species are better adapted to this system than others. For technical details on aquaponic nutrient delivery, see Recirculating Aquaculture Tank Production Systems: Aquaponics—Integrating Fish and Plant Culture (Rakocy et al., 2006).
Plants Adapted to Aquaponics: The selection of plant species adapted to hydroponic culture in aquaponic greenhouses is related to stocking density of fish tanks and subsequent nutrient concentration of aquacultural effluent. Lettuce, herbs, and specialty greens (spinach, chives, basil, and watercress) have low-to-medium nutritional requirements and are well adapted to aquaponic systems.
Plants yielding fruit (tomatoes, bell peppers, and cucumbers) have a higher nutritional demand and perform better in a heavily stocked, well-established aquaponic system. Greenhouse varieties of tomatoes are better adapted to low-light, high-humidity conditions in greenhouses than field varieties are.
Fish Species: Several warm-water and cold-water fish species are adapted to recirculating aquaculture systems, including tilapia, salmon, trout, perch, Arctic char, and bass. However, most commercial aquaponic systems in North America are based on tilapia. Tilapia is a warm-water species that grows well in a recirculating tank culture. Furthermore, tilapia is tolerant of fluctuating water conditions such as pH, temperature, oxygen, and dissolved solids. Tilapia produces a white-fleshed meat suitable to local and wholesale markets. The literature on tilapia contains extensive technical documentation and cultural procedures. In Australia, Barramundi and Murray cod fish species are raised in recirculating aquaponic systems.
Water-Quality Characteristics: Fish raised in recirculating-tank culture require good water-quality conditions. Water-quality testing kits from aquacultural supply companies are fundamental. Critical water-quality parameters include dissolved oxygen, carbon dioxide, ammonia, nitrate, nitrite, pH, chlorine, and other characteristics. The stocking density of fish, growth rate of fish, feeding rate and volume, and related environmental fluctuations can elicit rapid changes in water quality; constant and vigilant water-quality monitoring is essential.
Biofiltration and Suspended Solids: Aquaculture effluent contains nutrients, dissolved solids, and waste byproducts. Some aquaponic systems are designed with intermediate filters and cartridges to collect suspended solids in fish effluent, and to facilitate conversion of ammonia and other waste products to forms more available to plants, prior to delivery to hydroponic vegetable beds. Other systems deliver fish effluent directly to gravel-cultured hydroponic vegetable beds. The gravel functions as a “fluidized bed bioreactor,” removing dissolved solids and providing habitat for nitrifying bacteria involved in nutrient conversions. Design manuals and technical documentation linked from the Resources section can help growers decide which system is most appropriate.
Component Ratio: Matching the volume of fish-tank water to the volume of hydroponic media is known as component ratio. Early aquaponics systems were based on a ratio of 1:1, but 1:2 is now common and tank: bed ratios as high as 1:4 are employed. The variation in range depends on type of hydroponic system (gravel vs. raft), fish species, fish density, feeding rate, plant species, etc. Further, in shallow-bed systems where only three inches in depth are employed for the production of specialty greens such as lettuce and basil, the square footage of grow space will increase four times. Depending on the system design, the component ratio can favor greater outputs of either hydroponic produce or fish protein. A “node” is a configuration that links one fish tank to a certain number of hydroponic beds. Thus, one greenhouse may contain multiple fish tanks and associated growing beds, each arranged in a separate node.
The University of the Virgin Islands Aquaponic System
James Rakocy, Ph.D. and associates at the University of the Virgin Islands (UVI) have worked on developing a commercial-scale aquaponic system for more than thirty-eight years. Rakocy, now an aquaponics consultant, sees integrated water-reuse systems as a viable solution to sustainable food production, especially in developing countries and arid regions – such as the Caribbean Islands – where fresh water is scarce.
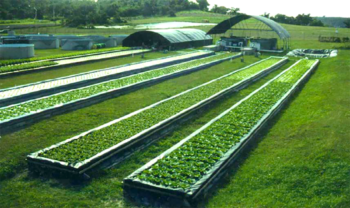
UVI aquaponic system. Photo: University of the Virgin Islands
In the Rakocy system, Nile and red tilapia are raised in fish-rearing tanks, and the aquacultural effluent is linked to floating raft hydroponics. Basil, lettuce, okra, and other crops have been raised successfully, with outstanding quality and yields.
The system components are as follows: four fish-rearing tanks at 2,060 gallons each, clarifiers, filter and degassing tanks, air diffusers, sump, base addition tank, pipes and pumps, and six hydroponic troughs totaling 2,303 square feet (Aquaponic Doctors, no date). The pH is monitored daily and maintained at 7.0 to 7.5 by alternately adding calcium hydroxide and potassium hydroxide to the base addition tank, which buffers the aquatic system and supplements calcium and potassium ions at the same time. The only other supplemental nutrient required is iron, which is added in a chelated form once every three weeks.
Tilapia are stocked at a rate of 77 fish per cubic meter for Nile tilapia, or 154 fish per cubic meter for red tilapia, and cultured for 24 weeks. The production schedule is staggered so that one tank is harvested every six weeks. After harvest, the fish tank is immediately restocked. The fish are fed three times daily with a complete, floating fish pellet at 32% protein. Projected annual fish production is 4.16 metric tons for Nile tilapia and 4.78 metric tons for red tilapia.
In one notable experiment, the UVI researchers compared the yields of a leafy herb (basil) and a fruiting vegetable (okra) grown in aquaponic vs. field production systems. Basil and okra were raised in raft hydroponics. Yields of aquaponic basil were three times greater than field-grown, while yields of aquaponic okra were 18 times greater than field-grown. Based on a market price in the U.S. Virgin Islands of $22 per kilogram for fresh basil with stems, researchers calculated gross income potential. The aquaponic method would result in $515 per cubic meter per year, or $110,210 per system per year. This compares to field-produced basil at $172 per cubic meter per year, or $36,808 per year, for the same production area. When fish sales are included, the aquaponic system yields $134,245 (Rakocy et al., 2004).
The UVI research team offers a short course on aquaponics each year at the UVI agricultural experiment station to provide in-depth technical support. The course is the premier educational training program available to farmers worldwide.
Aquaponics and Aquaculture Program
See also: Aquaponics Systems
Especially see:
Update on Tilapia and Vegetable Production in the UVI Aquaponic System. By James E. Rakocy, Donald S. Bailey, R. Charlie Shultz, and Eric S. Thoman. Pages 676-690. In: New Dimensions on Farmed Tilapia: Proceedings of the Sixth International Symposium on Tilapia in Aquaculture, held September 12-16, 2004 in Manila, Philippines.
Proceedings paper (15 pages)
PDF Presentation (49 pages)
Aquaponic Production of Tilapia and Basil: Comparing a Batch and Staggered Cropping System. By James E. Rakocy, R. Charlie Shultz, Donald S. Bailey, and Eric S. Thoman. Agricultural Experiment Station, University of the Virgin Islands.
Economics and Business Planning for Aquaponics
A combined recirculation aquaponic system incorporating fish and vegetables has been shown to provide both financial and environmental benefits in some research studies and commercial applications. The sharing of resources (i.e., water and nutrients) can result in a reduction of fertilizer and waste-water discharge, as well as reduced water use in vegetable production. Aquaponic production can be feasible but requires a large capital outlay and annual operating costs in excess of $100,000 in successful systems (Holliman et al., 2008).
Commercial aquaponics is still relatively new, and the most successful business models tend to be larger operations. Greenfeld et al. (2018) note that in addition to scale considerations (where larger systems have an economic edge), profitability is significantly determined by retail prices and improved business plans. Successful producers pay keen attention to financial planning and risk management, understand the place of consumer perception (and subsequent willingness to pay more for added value), and communicate the environmental benefits of aquaponic systems to their customers.
However, aquaponics can be a good fit for beginning farmers of small to medium scale who really think through their marketing plans and understand what it takes to sell their product. Successful aquaponics operations are complex businesses that require a high degree of planning, and the potential for failure is real. “There is a theme among producers who fail in aquaponics,” says Kevin Heidemann, a former Kentucky State University graduate student who conducted an extensive economic study on aquaponics (see Heidemann, 2015). “Start-up costs can range from $1 million to $1.5 million … to sell lettuce! [Let that sink in…]. Marketing is often an afterthought, but profitable producers have thought of that first” (Heidemann, 2018). Although land and farm-equipment costs are generally lower for aquaponics operations (you won’t need much land or a tractor with an assortment of implements) you will need some specialized equipment, including tanks, recirculation piping and pumps, lighting, grow beds, and climate-control technology, in addition to a greenhouse if you do not live in a sub-tropical region.
Operating costs can be high, as well, and your budget will have to consider fish fingerlings and vegetable starts, processing costs, utilities for heating, labor, and especially fish feed. In most operations, aquaponic producers realize profitability for the vegetable component, but profitability for both fish and vegetables is more difficult to achieve. Expect the fish component of an aquaponic operation to have the most operating costs (for feed and energy), but also the lowest income potential. Because the fish component of the system usually has the highest costs in an aquaponic system, the vegetable portion, due to crop nutrient requirements, may dictate the size of the fish component of the operation. For this reason, the cost ratio of fish to vegetables is of utmost concern. Successful aquaponic farms typically maintain good fish-to-vegetable cost ratios and market high-value fish and vegetables to higher-end markets to maintain profitable returns on both components. Thus, a solid marketing plan is crucial, and it’s not surprising to find that as the commonality among successful aquaponic producers.
Small-Scale Aquaponics
A small-scale aquaponic system can augment a home garden and provide a healthy source of food. If a production-scale operation is not what you’re are looking for, but you envision a small project for your homestead, backyard, or small farm, see Principles of Small-Scale Aquaponics from the Southern Regional Aquaculture Center.
Given the importance of marketing and selling, and the high start-up and operating costs an aquaponics operation will accrue, you’ll need to drill down and consider some practical questions that will help you determine if an aquaponics operation is feasible for you. One of the best ways to do this is to develop a comprehensive business and marketing plan. Some questions to ask are:
- What are the regulatory requirements you’ll need to consider? (see Licenses and Permits below)
- Who are your potential customers and are they easily accessible?
- Are your products unique and/or of high quality? Is there a high demand?
- Do you have the option to sell high-quality fish to a reliable market for a justifiable price?
- Are fresh vegetables currently accessible to your market? If so, what kind of vegetables are grown in your area? At what volume and price and during what season?
A recent global study found that the majority of 257 surveyed aquaponics farms lost money (Bosma et al., 2017). Thus, the ability to market high-value products from aquaponic systems cannot be over-emphasized. The most profitable aquaponics operations have tended to be in areas where fresh vegetables are expensive, and where consumers are used to paying higher prices (Engle, 2015). For many reasons, aquaponics is a risky endeavor. Developing a competitive and realistic marketing strategy, as well as establishing a workable fish-to-vegetable cost ratio, are key in determining profitability in aquaponic production.
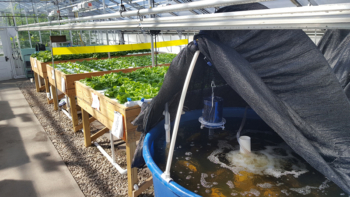
Aquaponic system. Photo: University of New Hampshire Experiment Station
Your plan should be as realistic as possible and take all costs into account. The best way to write a comprehensive plan and budget is to look at what other successful operators have done and build from there. Heidemann (2015) wrote an extensive study of three commercial aquaponics systems in Texas, Florida, and the U.S. Virgin Islands, which can be useful in developing a budget for a new operation. It contains detailed budgets, including construction and start-up costs, operating costs, and revenue figures. For more information, see:
- Commercial Aquaponics Case Study #1: Economic Analysis of Lily Pad Farms. 2015. By Kevin Heidemann.
- Commercial Aquaponics Case Study #2: Economic Analysis of Traders Hill Farms. 2015. By Kevin Heidemann.
- Commercial Aquaponics Case Study #3: Economic Analysis of the University of the Virgin Islands Commercial Aquaponics System. 2015. By Kevin Heidemann.
“The few studies developed to date show good potential for aquaponically produced vegetables to be profitable, with the fish portion possibly breaking even or incurring a net loss. Premium prices in high-end markets will be necessary for aquaponically produced vegetables and fish to be profitable. Additional costs and risks associated with these complex systems must be analyzed carefully before investing in aquaponics” (Engle, 2015).
Licenses and Permits
Prospective aquaponic operators should be aware of the types of permits or licenses that may be required in their states and municipalities. “State and local authorities generally regulate activities and issue permits for zoning, building, land, and water use. They also oversee waste discharge and aquaculture production practices and species” (Rumley, 2010). Depending on the resource concern, agencies establish permitting procedures to ensure that water resources, invasive species, and human-health concerns are addressed.
Your county Cooperative Extension Educator or State Aquaculture Coordinators (see Resources below) can very likely give you some good advice on where to start when thinking about permitting for aquaponics. Similar to land-based value-added agriculture, aquaponic production and sales will require systems to address food safety, natural resource conservation, on-farm processing and marketing of products, and the permitting of buildings and structures.
Table 3. Permits | ||
Possible Permit Types, depending on your state | Authority | Notes, Resources |
Business license, DBA, business structure | Local or municipal | Farmers’ Guide to Business Structures |
Food safety | State and/or local health department | HACCP plan |
FDA | Food Safety Modernization Act – Produce Safety Rule; GAP Certification (produce). ATTRA Food Safety page. | |
On-farm processing | State or local departments of health and/or agriculture | Food safety, weights and measures, labeling |
Selling agricultural products | State department of agriculture or department of natural resources | Dealer license for selling seafood |
Permit for raising fish | State game commission | Including permits for invasive species |
Building permits | Local or municipal | Greenhouses, foundations, etc. |
Effluent discharge | US EPA, Clean Water Act, National Pollutant Discharge Elimination System; State department of natural resources or conservation | Depending on the type of system, the amount of fish harvested, and the amount of feed used |
Water supply and use | Local water district | Well permitting, ground water regulations |
Evaluating an Aquaponic Enterprise
Aquaponics is an integrated food-production system that is more complicated than hydroponics, requires more training, and exhibits greater marketing challenges. Transitioning to a more ecological and sustainable production system necessitates a mind-change in the hydroponics business community. As this publication suggests, aquaponics can be done efficiently because it moves to the next level, a level where waste becomes integral to the system and the where the microbial component is critical for providing plant fertility.
Currently there is a bifurcation in the aquaponics industry. There are the big players (e.g., Superior Fresh in Wisconsin), and small to mid-scale operations (e.g., Cabbage Hill Farm in New York), and even smaller operations that rely on recycled IBC totes and barrels, including do-it-yourself technologies propagated on YouTube and elsewhere. These scales of operation are very different. Whether you are a beginning farmer investigating aquaponics as an enterprise or an established farmer seeking to scale up, there are some important factors to consider before incurring the expense of setting up a system.
First, it’s important to know that in aquaponics, you are dealing with two different life forms that need to be managed at peak health through a variety of constantly changing conditions (e.g., pH and temperature). You’ll need to consider the size of the operation you can feasibly handle, and you’ll have to figure out the permitting process. There is a very steep and long learning curve in starting an aquaponic operation. Most beginners drastically underestimate how difficult operating a multitrophic system can be, and managers must be consistently at top of their game. Importantly, do not go into aquaponics quickly. Instead, consider spending a year with a smaller system to learn, and take advantage of all the mistakes you’re sure to make. When you’ve gained some hands-on experience, you can write a truly realistic business and marketing plan and be in a much better position to be profitable in aquaponics (Filipowich, 2018).
Jonathan Van Senten, a Research Scientist in Aquaculture Economics and Marketing at Virginia Tech Agricultural Research and Extension Center, gives sound advice for those wanting to begin an aquaponic operation (2018). First, be conservative in estimating costs and prices when developing your business plan. Take into consideration the costs for initial investment, such as land, buildings and greenhouses, depreciation, and taxes. Production costs (fish stocks and seedlings, feed, disease control, labor, fuel) can vary, and then there are the costs associated with processing and marketing. The SARE case studies referenced above can be a huge help in estimating your start-up and operating costs.
Second, have a thorough understanding of what it is you’re selling. You may have an idea of what your business is about and the products you offer, but not everyone sees the same thing. You might be too close to your product and look at it from your own perspective. Take a holistic look at your operation with new eyes, and get a sense of what the public sees when you offer products for sale. You may be selling lettuce and tilapia, but what about the less tangible aspects of your offerings that tell a story and can connect with your customer? Perhaps you can market the local and sustainable aspect of your operation.
Third, keep good and accurate financial statements. Aquaponics, as has been stated, is complex, and understanding your cash flow, operating expenses, and depreciation can help you make small changes to be more efficient and productive.
Finally, think about what are you hoping to get out of your operation. What are your goals? Are you interested in full-time work to support a family? Is addressing food insecurity and/or sustainability while breaking even on the operation most important to you? Whatever your goal, start small and don’t put too much at risk until you understand the system and the numbers. Give yourself a year or two with a smaller system to make mistakes and learn—then you’ll be in a much better position to scale up.
Building and equipping a commercial-sized aquaponic greenhouse can cost in excess of $30,000, depending on the system design and choice of components. Due to the highly technical nature of aquaponics and the expense associated with greenhouse production, prospective growers are advised to thoroughly investigate production methods and market potential. Sequential considerations and learning opportunities geared to evaluating an aquaponic greenhouse enterprise are listed below.
- Aquaponic greenhouses yield two food products. To evaluate greenhouse profitability, obtain typical yields and market prices for hydroponic vegetables and fish, and investigate local and regional markets and related points of sale. Retail sales directly out of your greenhouse or roadside stand might be an ideal situation, but this will depend on your location.
- Aquaponics is one method of hydroponics, and hydroponics is one method of greenhouse production. Consider lower-cost and simpler alternatives. Bag culture of greenhouse vegetables — raising plants in polyethylene grow bags filled with compost-based potting mixes — is a simple and productive way to get started in greenhouse vegetable production. You may quickly find that your biggest challenge is weekly marketing of fresh produce, rather than successful production of vegetables. This includes labor to harvest vegetables, grading and packing with brand-name labels, post-harvest handling methods to maintain superior quality, and quick delivery of perishable produce to established markets.
- Read technical and popular literature on recirculating aquaculture and aquaponics to become familiar with production methods, yields, and market prices for fresh fish and hydroponic vegetables. The Resources listed below provide quick access to reading material, diagrams and images, and related details.
- Visit an aquaponic greenhouse for firsthand observations. Take lots of pictures to document the system components and how they relate to one another. Keep in mind that aquaponic growers are busy people with a considerable investment in time and resources to establish their businesses.
- Attend a short course. One of the most notable is the course offered by the University of the Virgin Islands. Nelson and Pade, Inc. has a list of training courses and seminars. In addition, aquaculture societies and associations usually offer conferences and workshops that cover many aspects of aquaponic production. See Aquaculture and Aquaponic Organizations and Journals in the Further Resources section below for more details.
- Obtain one or two aquaponic training manuals to provide detailed technical specifications. The book Aquaponic Food Production: raising fish and plants for food and profit; the Desktop Aquaponics Booklet; and an Aquaponics Curriculum from Nelson and Pade, Inc. are good starting points. When you are ready to explore a commercial system, the Guide to Aquaponics from Joel Malcolm’s Backyard Aquaponics in Western Australia contains in-depth technical specifications and illustrations. For more information on accessing these training manuals, see the Further Resources section below.
- Hire an agricultural consultant for expert advice and consultation, and to shorten the time and risk involved in getting started. A few consultants with expertise in aquaponics are listed in the Further Resources section below.
- Lastly, avoid the “inventor’s urge” to reinvent the wheel. Successful aquaponic greenhouse operators have already figured out the system components and methods of production, based on years of research and experience. Pick one of the existing models and duplicate it insofar as possible. The old saying, “Get the engine running first, then adjust the carburetor,” can be aptly applied to aquaponic start-up greenhouses.
References
Aquaponic Doctors. No date. James Rakocy bio.
Bosma, Roel H., Ysette Lacambra, Ynze Landstra, Chiara Perini, Joline Poulie, Marie J.Schwaner, and Yi Yin. 2017. The financial feasibility of producing fish and vegetables through aquaponics. Aquacultural Engineering. Volume 78, Part B. p. 146-154.
Burden, Dan, and D. Allen Pattillo. 2018. Aquaponics. Agricultural Marketing Resource Center. Iowa State University.
Engle, Carole R. 2015. Economics of Aquaponics. Southern Regional Aquaculture Center.
Filipowich, Brian. Aquaponics Association. Personal communication, 2018.
Greenfeld, Asael, Nir Becker, Jennifer McIlwain, Ravi Fotedar, and Janet F. Bornman. 2018. Economically viable aquaponics? Identifying the gap between potential and current uncertainties. Reviews in Aquaculture, 1–15.
Heidemann, Kevin. 2015. Economic Analysis of Commercial Aquaponic Production Systems. SARE Project Final Report GS13-125.
Heidemann, Kevin. University of Kentucky SARE Project Director. Personal communication, 2018.
Holliman, J.B., J. Adrian, and J.A. Chappell. 2008. Integration of Hydroponic Tomato and Indoor Recirculating Aquaculture Production Systems: An Economic Analysis. Special Report No. 6. Alabama Agriculture Experiment Station. Auburn University.
Khiari, Zied, Soba Kaluthota, and Nick Savidov. 2019. Aerobic bioconversion of aquaculture solid waste into liquid fertilizer: Effects of bioprocess parameters on kinetics of nitrogen mineralization. Aquaculture. Vol. 500. p. 492–499.
NHPR. 2018. The Future of Food? Aquaculture & Aquaponics. New Hampshire Public Radio, November 20.
Rakocy, James E., Michael P. Masser, and Thomas M. Losordo. 2006. Recirculating Aquaculture Tank Production Systems: Aquaponics—Integrating Fish and Plant Culture. Southern Regional Aquaculture Center.
Rumley, Elizabeth R. 2010. Aquaculture and the Lacey Act. National Ag Law Center, University of Arkansas.
Savidov, Nick. 2016. Agro-Ecology in Aquaponics. Presentation for NOA Fisheries Course, Lethbridge College, Ontario.
Savidov, Nick. Lethbridge College Aquaponics Researcher. Personal communication, 2018.
Van Senten, Jonathan. Research Scientist in Aquaculture Economics and Marketing at Virginia Tech Agricultural Research and Extension Center. Personal communication, 2018.
Further Resources
Agricultural Consultants for Integrated Hydroponics and Aquaculture
Brian Filipowich
Anacostia Aquaponics
Washington, DC
Dr. James Rakocy and Dr. Wilson Lennard
The Aquaponics Doctors
Fisheries Technology Associates, Inc.
506 Wabash Street
Fort Collins, CO 80522-3245
970-225-0150
Nelson and Pade, Inc.
P.O. Box 761
Montello, WI 53949
608-297-8708
Pentair Aquatic Eco-Systems, Inc.
400 Regency Forest Drive, Suite 300
Cary, NC 27518
407-543-1204
The Aquaponic Source
5151 Ward Rd, Unit 3
Wheat Ridge, CO 80033
303-720-6604
Alternative Aquaculture Feeds
Aquaculture feeds: addressing the long term sustainability of the sector. 2012. By A.G.J Tacon, M.R. Hasan, G. Allan, A,-F. El-Sayed, A. Jackson, S.J. Kaushik, W.-K. Ng, V. Suresh, and M.T. Viana. 2012. In: R.P. Subasinghe, J.R. Arthur, D.M. Bartley, S.S. De Silva, M. Halwart, N. Hishamunda, C.V. Mohan and P. Sorgeloos, (eds.). Farming the Waters for People and Food. Proceedings of the Global Conference on Aquaculture 2010, Phuket, Thailand. FAO, Rome and NACA, Bangkok.
[From the abstract:] The current discussion about the use of marine products as aquafeed ingredients focuses on fishmeal and fish oil resources, the sustainability of the aquaculture sector is more likely to be linked with the sustained supply of terrestrial animal and plant proteins, oils and carbohydrate sources for aquafeeds, particularly so because a significant proportion of aquaculture production is of non-carnivorous species. Therefore, aquaculture-producing countries should place more emphasis on maximizing the use of locally available feed-grade ingredient sources and move away from the use of potential food-grade feed resources.
Alternative Protein Sources for Aquaculture Feeds. 2012. By Ferouz Y. Ayadi, Kurt A. Rosentrater, and Kasiviswanathan Muthukumarappan. Journal of Aquaculture Feed Science and Nutrition. Vol. 4, No.1. p. 1-16.
[From the abstract:] Reviews alternative protein sources and looks at problems and advantages of animal byproducts, fishery byproducts, bacterial proteins, and plant proteins, and discusses nutrient values and rates of feeding.
Feeding aquaculture in an era of finite resources. 2009. By Rosamond L. Naylor, Ronald W. Hardy, Dominique P. Bureau, Alice Chiu, Matthew Elliott, Anthony P. Farrell, Ian Forster, Delbert M. Gatlin, Rebecca J. Goldburg, Katheline Hua, and Peter D. Nicholsi. Proceedings of the National Academy of Sciences. Vol. 106, No.36. p. 15103–15110.
[From the abstract:] This article reviews trends in fishmeal and fish oil use in industrial aquafeeds, showing reduced inclusion rates but greater total use associated with increased aquaculture production and demand for fish high in long-chain omega-3 oils. With appropriate economic and regulatory incentives, the transition toward alternative feedstuffs could accelerate, paving the way for a consensus that aquaculture is aiding the ocean, not depleting it.
Feed Matters: Satisfying the Feed Demand of Aquaculture. 2015. By Albert G.J. Tacon and Marc Metian. Reviews in Fisheries Science & Aquaculture. Vol. 23. p. 1–10.
[From the abstract:] This paper attempts to make a global analysis of aquaculture growth, its role in global food production, and to update the estimates of compound feed dependent fish and crustacean species.
Green Feed In The Marine Fish Farming: How to communicate water benchmarks to stakeholders. 2009. By Therese A. M. Jansson. Swedish University of Agricultural Sciences, Department of Economics, Degree Thesis in Business Administration.
[From the abstract:] The use of unsustainable (limited) and expensive fishmeal and fish oil should be curtailed. The challenge is to identify more environmentally friendly and cheap substitutes for the unsustainably fishmeal and fish oil. Several trials have been made to reduce the quota of unsustainable fishmeal in farming the deep blue, and where e.g. feed has been substituted to one extend by soybean meal. Nonetheless, it is crucial that the substitute for fishmeal and fish oil maintain both the quality and quantity of production that the original products achieve.
Microalgae in aquafeeds for a sustainable aquaculture industry. 2017. By Mahfuzur Rahman Shah, et al. Journal of Applied Phycology.
[From the abstract:] This comprehensive review summarizes the most important and recent developments of microalgae use as supplement or feed additive to replace fishmeal and fish oil for use in aquaculture.
n-3 Oil sources for use in aquaculture – alternatives to the unsustainable harvest of wild fish. 2008. By Matthew R. Miller, Peter D. Nichols, and Chris G. Carter. Nutrition Research Reviews. Vol. 21, No. 2. p. 85–96.
[From the abstract:] The benefits and challenges involved in changing dietary oil in aquaculture are highlighted and four major potential sources other than fish oil, are compared. These sources of oil contain precursors to key essential fatty acids, and include (1) other marine sources of oil; (2) vegetable oils that contain biosynthetic precursors, such as stearidonic acid, (3) single-cell oil sources, (4) vegetable oils derived from oil-seed crops that have undergone genetic modification to contain n-3 LC-PUFA.
Utilization of waste from a marine recirculating fish culture system as a feed source for the polychaete worm, Nereis virens. 2011. By Nicholas Brown, Stephen Eddy, and Stefanie Plaud. Aquaculture. Volumes 322–323, No. 21. p. 177-183.
[From the abstract:] The polychaete worm was fed with wastes from a fish culture system and grew well on these wastes and valuable protein and lipids were recovered. The results demonstrate that production of N. virens using fish wastes is highly efficient. This species is an excellent candidate for integrated aquaculture and waste recycling and may offer economic and environmental benefits.
Aquaponics and Aquaculture Videos
Aquaponics Videos. By Ben Boothe.
Includes more than 80 videos on all aspects of aquaponics and vegetable and fish culture.
Aquaponics YouTube Playlist. Iowa State University.
Instructional videos on nutrients, biofiltration, aeration, UV light sterilization, vegetable production, fish culture, pond management, and how to get started.
U.S. Aquaculture Society Webinar Series
Developed by the National Aquaculture Association and the North Central Regional Aquaculture Center, with support from the United Soybean Board, these webinars provide information on everything from starting an aquaponics operation to health and biosecurity to business planning.
Aquaculture and Aquaponics Libraries and Resource Lists
Aquaponics. Alternative Farming Systems Information Center, USDA National Agricultural Library
National Agricultural Library is one of four national libraries of the United States and houses one of the world’s largest collections devoted to agriculture. The section on aquaponics has extensive links to aquaponic start-up and management resources.
Aquaculture Library. National Oceanic and Atmospheric Administration Aquaculture Program.
Online library for aquaculture-related material including policies, newsletters, and fact sheets.
Environmentally Friendly Aquaculture Digital Library
National Sea Grant Library
The National Sea Grant Library (NSGL) contains a complete collection of Sea Grant-funded work. The NSGL maintains a bibliographical database containing more than 36,000 records that can be searched by author or keyword, or browsed by topic. Selected items include proceedings from recirculating aquaculture conferences and related documents. The Environmentally Friendly Aquaculture Digital Library is a topic-oriented portal to NSGL, organized by subject category.
Aquaculture and Aquaponic Farms
Anything Aquaponics
One of the most comprehensive lists of farms and organizations dedicated to providing food to communities through aquaponics. Includes links to educational videos, community partners, and design/consultation services.
Superior Fresh
Superior Fresh produces 160,000 pounds of Atlantic salmon and 1.8 million pounds of leafy greens annually under 40,000 square feet of fish house space and 123,000 square feet of greenhouse, with zero discharge of production water.
Appendix I: Organic Aquaculture/ Aquaponics
Organic production of crops and livestock in the United States is regulated by the Department of Agriculture’s National Organic Program, or NOP. The NOP is an organic certification and marketing program that ensures foods and food products labeled as “organic” meet universal standards and guidelines for organic production. Inputs used in organic production — such as feed and fertilizers — must be of natural origin and free of synthetic materials. A farm plan, documentation of inputs and production methods, and farm inspection are required to obtain certified organic status. This process allows farm products to be labeled and sold as organic.
Organic trout, tilapia, salmon, and other fish species are raised in Europe, Australia, and Israel using production standards developed by international organic certification agencies (for more information see IFOAM Norms for Organic Production and Processing, Version 2014. However, organic aquaculture was not clearly defined in the NOP regulations and the lack of organic aquaculture guidelines has hampered the growth of a domestic organic aquaculture industry in the United States.
To address the issue of organic aquaculture, the National Organic Standards Board (NOSB) established an Aquatic Animals Task Force in June 2000. In 2003, a second group, The National Organic Aquaculture Working Group (NOAWG), formed to provide further guidance and clarification to the NOSB. The report published by NOAWG provided proposed recommendations on organic aquaculture standards to NOSB (see Lockwood et al., 2005).
Hydroponics, aquaponics, and aeroponics have, however, been eligible for certification under the National Organic Program. There has been some controversy over these soil-less production systems, and some organic advocates have called for their prohibition. In 2010, the NOSB sent a recommendation to the National Organic Program (NOP) that stated “growing media shall contain sufficient organic matter capable of supporting natural and diverse soil ecology. For this reason, hydroponic and aeroponic systems are [should be] prohibited.” In 2015, noticing some ambiguous points in the report, the National Organic Program authorized a task force to explore how hydroponic and aquaponic production practices aligned with the USDA organic regulations (USDA, 2016). The NOSB made final recommendations to the NOP after a controversy-filled 2017 meeting where inclusion of hydroponic, aquaponic, and aeroponic systems in organic certification was brought to a vote. The motion to prohibit hydroponic and aquaponic production was defeated, but the motion to prohibit aeroponic production in organic certification passed and went on to the NOP for action (USDA, 2018).
Much like hydroponics, aeroponics is a soilless growing system that delivers water and nutrients directly to plant roots. Aeroponic systems differ in that they use an atomized spray to mist plant roots, instead of circulating water through the growing bed or channel.
As of this writing, there are still no clear guidelines for certification of aquaculture operations in the NOP regulations. However, certification is being conducted for aquaponic operations under the general rules that govern organic operations. As the NOSB, growers, industry representatives, and now the NOP discuss and debate the merits and concerns of organic aquaculture, hydroponics, and aquaponics, the number or certified aquaponic operations has continued to increase. A 2010 USDA Survey to Certifiers noted that in the United States, eight certifiers certified hydroponics, with 39 certifying hydroponic operations. When the survey was again conducted in 2016, there were 17 certifiers that certified hydroponic and aquaponic operations, while 30 certified hydroponic operations, 22 certified aquaponic operations, and a total of 69 certified container-based operations (McEvoy, 2016).
Appendix I References
Lockwood, George, Richard Nelson, and Gary Jensen (eds.). 2005. National Organic Aquaculture Working Group White Paper, Proposed National Organic Standards for Farmed-Aquatic Animals and Plants (Aquaculture). National Organic Aquaculture Working Group.
McEvoy, Miles V. 2016. Presentation to the National Organic Standards Board Meeting, April 25. USDA Agricultural Marketing Service, National Organic Program.
USDA. 2016. Hydroponic and Aquaponic Task Force Report, Memorandum to the NOSB. USDA AMS NOP.
USDA. 2018. Status of Organic Hydroponics, Aquaponics, Aeroponics; National Organic Standards Board Fall 2017 Updates. USDA Agricultural Marketing Service, National Organic Program.
Appendix II: Aquafeeds and Their Alternatives
Aquaponics has the ability to produce large quantities of sustainably grown, locally sourced food. With the right technology and monitoring it can be a zero-waste system that recycles nutrients in a closed loop, reducing the need to use resource-extractive soluble nutrients to fertilize crops. Much work needs to be done to close the loop, and if aquaponics producers can adopt technologies such as anaerobic bioreactors for recycling solid fish wastes (Khiari et al., 2019), and utilize sustainably sourced feedstuffs (NOAA, 2012), the industry will truly approach the zero-waste system to which it aspires.
Whereas fertilizer inputs to the plant component of the aquaponic system are eliminated and replaced by nutrients from the fish, the fish component still relies on fed feed, which is a significant input, both economically and with respect to sustainability. Sourcing appropriate fish feeds has been a problem for the aquaculture industry for decades. Historically, fish meal and oil has been the principal protein source for farmed fish, given its amino acid profile (omega 3 and 6 fatty acids). The cost of fish meal and oil has increased mainly due to competition with the livestock feed industry, resulting in less fish meal and oil being used in the aquaculture sector (El-Sayed, 1999). The reduction of fish stocks (i.e. small forage fish like menhaden, anchovies, and sardines) for the manufacture of fish meal and fish oil is also a serious concern (Naylor et al., 2000; Rubicon Resources, 2018; and Allsopp et al., 2008), and researchers and practitioners are looking into alternative feeds including soybeans, barley, rice, peas, and other crops, along with canola, lupine, wheat gluten, corn gluten, various plant proteins, algae, and seafood processing co-products (NOAA, 2012). These products hold promise, especially when used in a mixed diet, to supply the nutritional needs of aquaculturally and aquaponically produced fish.
Gross Nutrient Value – Fish Meal vs. Unprocessed Ingredients | |||||
Ash | N | Protein | Fat | GE | |
Fish meal | 14 | 11 | 68 | 10 | 20 |
Blood meal | 3 | 13 | 84 | 0 | 21 |
Meat and bone | 33 | 8 | 53 | 7 | 16 |
Meat low ash | 3 | 13 | 81 | 10 | 26 |
Poultry meal | 14 | 9 | 57 | 17 | 21 |
Feather meal | 3 | 12 | 74 | 10 | 22 |
Soybean | 8 | 7 | 45 | 3 | 16 |
Lupin | 3 | 5 | 32 | 5 | 17 |
Field pea | 3 | 4 | 24 | 1 | 16 |
Cow pea | 3 | 4 | 22 | 2 | 16 |
What gluten | 1 | 12 | 72 | 0 | 22 |
Corn gluten | 1 | 9 | 58 | 0 | 22 |
Wheat | 1 | 2 | 11 | 2 | 17 |
Sorghum | 2 | 2 | 13 | 4 | 17 |
Source: Allen, 2015 |
Producing fish from plant-based proteins seems, at face value, to be a solution, especially for omnivorous species like carp and tilapia. Carnivorous fish like salmon can utilize some plant and algal-based feedstuffs but need long chain fatty acids that come from marine and terrestrial animal-based proteins. The constraints of plant-based sources include low digestibility, low omega 3 and 6 fatty acids, and anti-nutritional factors in plant-based proteins (see Naylor et al., 2000). For this reason, fish meal and oil will still play a small role in the manufacture of fish feeds (especially for salmon), but when coupled with alternative feedstuffs like poultry meal (Yones and Metwalli, 2016), algae (Hemaiswarya et al., 2011), and insect proteins (Newton et al., 2013), less pressure can be placed on wild capture fisheries, especially if fish wastes and bycatch can be used to supply some of the protein needs of aquaculturally produced fish (see Hardy et al., 1983 and de Arruda et al., 2007).
Choosing alternative aquaculture feeds can be a daunting process for aquaponics producers who aspire to the adoption of sustainable practices. Reducing fish meal and fish oil is important for the health and well-being of the world’s fisheries, and there are many alternatives, but research is needed to ensure adequate nutrition is provided to farm-raised species (Krogdahl, 2016).
Appendix II References
Allan, Geoff. 2015. Sustainable Aquaculture Feeds. Industry & Investment NSW, Port Stephens Fisheries Institute, Australia.
Allsopp, Michelle, Paul Johnston, and David Santillo. 2008. Challenging the Industry on Sustainability. Greenpeace International.
de Arruda, Lia Ferraz, Ricardo Borghesi, and Marilia Oetterer. 2007. Use of fish waste as silage – A review. Brazilian Archives of Biology and Technology Vol. 50, No. 5.
El-Sayed, Abdel-Fattah, M. 1999. Alternative dietary protein sources for farmed tilapia, Oreochromis spp. Aquaculture 179, 149–168.
Hardy, Ronald W., Karl D. Shearer, Fred E. Stone, and Dave H. Wieg. 1983. Fish Silage in Aquaculture Diets. Journal of the World Aquaculture Society, Volume14, Issue 1‐4.
Hemaiswarya, S., R. Raja, R. Ravi Kumar, V. Ganesan, and C. Anbazhagan. 2011. Microalgae: a sustainable feed source for aquaculture. World J Microbiol Biotechnol, 27:1737–1746.
Khiari, Zied, Soba Kaluthota, and Nick Savidov. 2019. Aerobic bioconversion of aquaculture solid waste into liquid fertilizer: Effects of bioprocess parameters on kinetics of nitrogen mineralization. Aquaculture. Vol. 500. p. 492–499.
Krogdahl, Ashild. 2016. The Fish Meal Dilemma – What are the Alternatives? 2016 World Nutrition Forum, Vancouver, Canada.
Naylor, Rosamond L., Rebecca J. Goldburg, Jurgenne H. Primavera, Nils Kautsky, Malcolm C. M. Beveridge, Jason Clay, Carl Folke, Jane Lubchenco, Harold Mooney, and Max Troel. 2000. Effect of aquaculture on world fish supplies. Nature, Vol 405.
Newton, G.L., D.C. Sheppard, and G.J. Burtle. 2013. Research Summary: Black Soldier Fly Prepupae – A Compelling Alternative to Fish Meal and Fish Oil. eXtension.
NOAA. 2012. The Future of Aquafeeds.
Rubicon Resources. 2018. 3 Promising Alternative Feeds for Aquaculture. Medium.
Yones AMM, Metwalli AA. 2016. Effects of Fish Meal Substitution with Poultry By-product Meal on Growth Performance, Nutrients Utilization and Blood Contents of Juvenile Nile Tilapia (Oreochromis niloticus). J Aquac Res Development 7:389.
Aquaponics – Multitrophic Systems for Sustainable Food Production
By Lee Rinehart, NCAT Agriculture Specialist
Published March 2019
© NCAT 2019
IP579
Slot 54
Version 032019
This publication is produced by the National Center for Appropriate Technology through the ATTRA Sustainable Agriculture program, under a cooperative agreement with USDA Rural Development. ATTRA.NCAT.ORG.