Building Healthy Pasture Soils
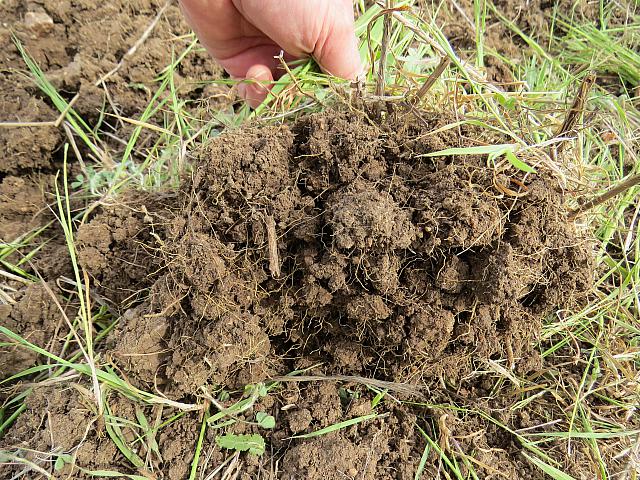
Photo: NCAT
By Lee Rinehart, NCAT Agriculture Specialist
Abstract
This publication, a supplement to ATTRA’s Managed Grazing Tutorial lesson on Pasture Fertility, introduces properties of soil, discusses evaluation and monitoring of soil quality, and introduces grazing management principles and techniques that promote healthy soil.
Introduction
Soil fertility is an ecosystem concept that goes well beyond a simple discussion of soil samples, fertilizers, and the nutrients needed to produce high yields. Regenerative grazing management and the inherent abilities of living, healthy soil promote productive pastures and animals and provide sustainable lifestyles for the people who work the soil.
Managing for soil health is holistic and complex, and involves regenerative, adaptive management, not mere conservation or relying on inputs of finite particles of fertility. With this type of management, we are observational and not reactive; we are looking at soil indicators such as soil aggregation and cover. We are looking for telltale signs of soil ill-health such as run-off, compaction, and bare ground.
Within a regenerative system, we are interested in feeding soil microorganisms with a constant diet of carbon from the sun. Soil microbes need habitat and a balanced diet. This is accomplished through plant diversity, living roots, and soil cover all year. The saying build it and they will come applies here… and if we make sure these microbes are fed, they will do the work of soil health and fertility for us.
Soil fertility is the common term used to describe the ability soil has to provide nutrients to plants at the right time. Traditionally, when soils are tested, a report is generated that recommends the appropriate amounts of nitrogen, phosphorus, and potassium, as well as micronutrients and lime, to supply a specific crop with all the nutrients it needs. This is the standard paradigm: one that reduces fertility to the presence and quantity of a few discrete elements. It has resulted in a system that has produced enormous yields, but has come at the cost of severe soil and biosphere degradation.
This publication looks beyond the standard paradigm to the underpinnings of what makes the system work as a whole: namely, the life in the soil and the environment that sustains it. Instead of a nutrient-based discussion, we will be looking at the relationship between grazing, soil ecology, and nutrient cycling, and we’ll consider some of the soil-management strategies farmers are adopting to enhance and sustain pasture productivity.
Let’s begin by looking at how the soil is put together and where all the processes occur. This environment, which I like to think of as a habitat, is characterized by physical, chemical, and biological processes. For example, think of a house being constructed. First, we have the physical structure (the foundation, studs, joists, and roof) that makes up the physical environment.
Next, there are the chemical processes that occur within this structure, which augment it and make it habitable, much like the plumbing, ventilation, and the electrical system. Finally, we have the biological element… the life that inhabits this structure and interacts with the environment, changing it to suit the inhabitant’s needs.
Properties of Soil
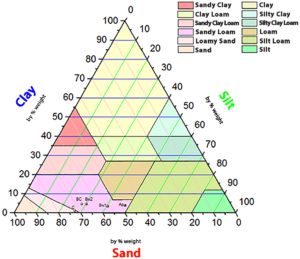
Figure 1: Soil Texture. Source: USDA NRCS
Physical Properties
In our construction analogy, from the foundation to the roof, the various parts are integral to the proper function of the house. Built on a foundation of soil particles, from the organic matter and roots that serve as the studs and nails to the roof constituted by the plants above ground, the system won’t work properly unless each part is fully functioning.
The soil’s physical properties describe how the various parts construct the environment that is inhabited by the plants and organisms. Through their interactions, the soil environment becomes a suitable habitat for sustained life. Like our house example, soil is constructed by various parts that contribute to its structure. The mineral fraction of the soil is like the foundation. It can be sand, silt, or clay and makes up about 45% of the soil volume. Pore space is about 50%, which is very important for water holding and air exchange. Organic matter makes up roughly 5% of the volume, but can range from 1% to 7% or more. Most agricultural soils are about 2% to 5% organic matter.
Soil texture, whether the soil is sand, silt, clay, or a combination of the three, and organic matter make up a very important soil physical property: soil structure. This structure is what gives soil its stability and makes it a resilient habitat for soil life, as well as preventing soil and nutrient loss through erosion and leaching.
Related to soil structure, and a very important physical indicator, is bulk density. Bulk density is a measure of pore space in the soil. Recall that pore space allows for water and air exchange, in addition to providing space for root growth. A good way to visualize bulk density is to think of the difference between a sponge and a brick. The sponge has pores and passages, whereas the brick is solid with no pore space. If these examples were soils, it’s easy to see which one would better accommodate root growth and air exchange.
Bulk density is expressed as the ratio of the weight of the soil to the volume of the soil, and is measured in grams per cubic centimeter. The lower the ratio, the more surface area, and the better the bulk density. As an example, soil humus has a bulk density of about 0.6 grams per cubic centimeter, and plants grow best when the bulk density is around 1.2. Compare that to concrete, which has a bulk density of 2.4.
To calculate the bulk density of your soil, you can access a nice fact sheet that has easy-to-follow instructions here.
Chemical Properties
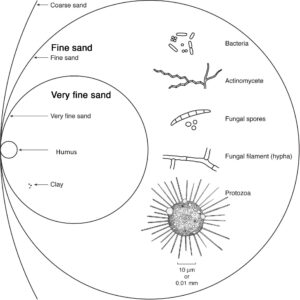
Figure 2: Particle Size. Source: NCAT
Chemical properties are what make the nutrient cycles happen. The soil composition and parent material, or rock origin, is the basis on which the chemical processes take place. Of particular importance to the chemical processes are soil pH and the size of the soil particles. These have an effect on the adsorption and release of nutrients for plant growth. Clay and humus particles provide the cation exchange capacity, or CEC, that is the storehouse for soil nutrients.
Biological Properties
“Lastly, we have the biological processes that involve microbiological activity, insects, earthworms, and small animals.” Plant roots provide habitat and release carbon sugars to feed soil microorganisms and contribute to the formation of glues, or glomalin, that bind soil into aggregates. And aggregates are the important soil structural component that provides microbiological habitat and gives soil its ability to withstand erosion and the leaching of nutrients.
Organic Matter, Soil Biology, and Nutrient Cycles
Plants need energy to grow and produce the crops we harvest, and we are all familiar with the way nature does this: through photosynthesis. We know that the energy used by plants comes from the sun and is transformed chemically inside the plant’s cells into sugars, and that the plant uses these sugars to power all its life processes, from stem elongation to grain filling. But what is less understood is exactly where photosynthate, the sugary product of photosynthesis, actually goes. Agronomists have found that anywhere from 20% to 70% of the sugars produced by plants never even make it to the plant parts above the soil surface, but instead are shunted to the roots to fuel root growth and, very importantly, to be oozed out of the roots into the surrounding soil. This sugar, root exudates, is the food that feeds the millions of microorganisms that constitute the soil rhizosphere.
Each organism contributes to the food web, whether it’s bacteria fixing nitrogen, protozoa and nematodes consuming other microbes and cycling nitrogen and carbon, or fungi, which have a particularly important role to play in the soil food web. There are basically two kinds of fungi: the decomposers, or saprophylic fungi, and those responsible for nutrient transfer, arbuscular mycorrhizal fungi. Arbuscular mycorrhizal fungi have a symbiotic relationship with plants through their hyphae, which form a sort of highway to facilitate nutrient transfer between plant roots and the surrounding soil, greatly increasing the ability of plant roots to access the soil profile. They also contribute to soil aggregate stability by producing glomalin, a glue that holds soil particles together. Soils with a lot of fungi are erosion-resistant and fertile. Practices that foster fungi associations in the soil will be covered below.
Through photosynthesis, carbon naturally cycles into the plant, where it’s converted into plant sugars. From there it goes to the roots and out into the soil, where root-sugar exudates feed soil microorganisms, in exchange for mineralized nutrients that are changed into a form that is available for plants to use. The microbes metabolize the sugars and respire carbon dioxide into the air, which ultimately ends up in the ocean or other water bodies. Managing carbon to keep it in the soil in the form of humus is the job of the farmer. The soil is the largest carbon sink that can be directly controlled by humans. Keeping the soil covered with growing vegetation for as much of the year as possible helps maintain carbon in the soil, through active photosynthesis and carbon cycling.
Soil carbon also comes from living things… stuff like plant roots, leaves, and stems and dead organisms. As these materials enter the soil, they become a part of the soil carbon pool and begin to cycle through the system, feeding first the microorganisms, and then plants as microorganisms die. Eventually, through the liquid carbon pathway, soil carbon comes to rest in a stable form called humus, which provides habitat for microorganisms and contributes to the nutrient- and water-holding capacity of the soil. Remember, a healthy soil consists of both the mineral fraction and the organic fraction. Soil humus makes up the complex, stable part of the organic fraction and gives the soil resiliency and stability.
Humus Formation in the Soil
According to soil scientist Christine Jones (2008; 2015), humus is created via the liquid carbon pathway, through resynthesized root exudates, which are mostly sugars. Carbon from organic-matter decomposition facilitates humification by feeding microbes, which feed plants with minerals. Plants then photosynthesize more and produce more roots, which produces more root-carbon root exudates, which goes into humus.
In a paper included in the Appendix, Dr. Christine Jones explains the soil liquid carbon pathway and how it is associated with nutrient movement.
Notice the plant root in the upper left-hand quadrant of the photo below. Plants interact with soil and soil organisms at the aggregate level, in the region known as the rhizosphere. Soil aggregates provide the structure and nutrient-holding capacity for plant growth, and are populated with bacteria, protozoa, fungi, nematodes, and arbuscular mycorrhizal fungi, which form the glues that hold the structure together.
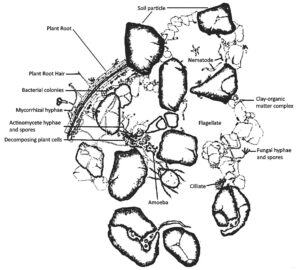
Figure 3: Soil Aggregate. Source: USDA
Mycorrhizal fungi form associations with the roots of many cash-crop, pasture, and cover-crop plants. They play a role in plant nutrient acquisition, are important in defense against certain soil-borne pathogens, and are involved in other vital processes such as water cycling and soil aggregation. With hyphae that connect the inside of the plant roots with the outside soil environment, these fungi form a symbiotic relationship with plants and serve as a sort of highway for the transport of important soil nutrients.
Mycorrhizal fungi form associations with a number of pasture species and cover crops, such as perennial grasses and legumes, cereal rye, wheat, barley, corn, and oats. However, they do not associate with brassicas, which include canola, rape, radish, buckwheat, beets, turnips, and mustard. These cover crops make excellent additions to a cover crop mix, and when planted with grasses and legumes, the mycorrhizal populations are not affected. However, some farmers plant a monoculture cover crop like daikon radish for the benefits of subsoiling from the deep roots, as well as the carbon they put back into the soil. Research by Charles White and Ray Weil has suggested that it takes about 60 days to re-populate a field with mycorrhizal fungi after growing a monoculture, non-mycorrhizal crop (2010).
Along with fungi, bacteria populate the soil profile by the billions. Not only are they the largest pool of nitrogen in the soil, but they come in various functional types, from decomposers to mutualists to pathogens. They are particularly abundant around plant roots, especially the root tips, and in green litter that is high in nitrogen, which they are breaking down to begin the nitrogen cycle. As decomposers, bacteria immobilize nutrients and help with nitrogen efficiency.
Nitrogen-fixing microbes include plant-associated bacteria and archaea. Biological nitrogen fixation accounts for around 65% of the nitrogen used by pasture and crop plants, and because 80% of the atmosphere consists of nitrogen, there is room for much more. We are most familiar with the rhizobium species that have a symbiotic relationship with plants, notably legumes, which obtain energy from the plant and convert nitrogen to plant-available forms. Archaea are less understood, but we know they are there. Green, growing plants have chlorophyll, which is part of a protein complex, and as Christine Jones, a soil scientist focusing on soil health, says, “Wherever you see green plants, there will also be an association with nitrogen-fixing bacteria or archaea” (2014).
Mycorrhizal fungi are also vitally important to the nitrogen-fixing process. They do not fix nitrogen, but transfer energy to nitrogen-fixing bacteria in the form of liquid carbon and to plants in the form of amino acid-bearing nutrients. Mycorrhizal fungi are a vital component of the “microbial bridge” between plants and soil nutrients.
Assessing Soil Quality
So, there are all these processes going on, and we’ve got to have a way to assess them. Chemical testing has long been the default approach farmers use to assess soil fertility, but we know that chemical analysis of soil nutrients is a finite, snapshot picture of plant-available nutrients at a moment in time. It doesn’t take into account the myriad processes that make those nutrients available in the first place. Today, we have some really good assessments that look at soil holistically and can help us understand the biology and how that biology can mineralize unavailable nutrients into plant-available nutrients. This in turn, can help us make decisions that enhance the functionality of our pasture soils.
A soil health assessment is concerned with how well the soil performs all of its functions. To do an assessment, we look at a series of indicators, or measurable properties, that can tell us how well the system is functioning and give insight into management practices for improvement.
Soil health indicators can be physical, chemical, and biological properties, processes, or characteristics of soils, as well as visual features of plants.
Physical indicators include bulk density, infiltration, soil structure, soil depth, and water-holding capacity. These characteristics relate to retention and transport of water and nutrients, habitat for soil microbes, crop productivity potential, compaction, plow pan, and water movement.
The chemical indicators include electrical conductivity, reactive carbon, soil nitrate, soil pH, and extractable phosphorus and potassium. All of these relate to biological and chemical activity, plant and microbial activity, and plant-available nutrients, as well as the potential for nitrogen and phosphorus loss.
And biological indicators are earthworms and other macro-organisms, microbial biomass carbon and nitrogen, particulate organic matter (small pieces of decomposing active organic matter), potentially mineralizable nitrogen, soil enzymes, soil respiration, and total organic carbon. These relate to microbial potential as a repository for carbon and nitrogen and soil productivity and nitrogen-supplying potential.
The NRCS Soil Health Assessment website has a series of fact sheets on the soil-quality indicators, as well as guidelines for assessing soil health.
Soil Testing and Monitoring
Getting a handle on all the soil processes that contribute to healthy soil seems to be a huge undertaking. It is not as easy as reading a chemical soil test report, though even those can be confusing at times. Fortunately, there are several assessments that are commercially available to test for soil health. Among these are the Cornell test, the Solvita test, and the Haney test. You can find more information on these tests below in the Resources section.
Soil Degradation and Regenerative Soil Management
Once you are familiar with the micro-structures that make up the larger soil environment, it becomes easier to understand the important role microorganisms play in soil fertility. In fact, they run the show. With this knowledge, we can better understand the soil-degradation spiral that has historically resulted in poor soil quality and the loss of soils through erosion.
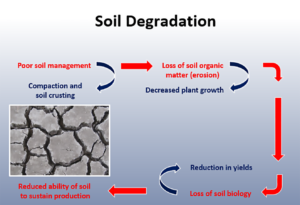
Figure 4: Soil Degradation. Source: NCAT
Soil-management techniques that have been taught and practiced for decades do harm to the soil environment and are directly responsible for soil loss. “Life support” technology has kept agriculture alive in spite of decreasing soil quality. Monocultures, extreme tillage, and bare-soil fallow have sent our soils on such a downward spiral that synthetic fertilizers and pesticides are needed, because the soil has nothing left to give.
Continuous tillage, lack of crop diversity, and poor grazing management (most notably lack of grazing-stock density and pasture rest) results in an unfavorable environment for soil organisms and consequently causes a loss of soil aggregation. The soil begins to compact and crust. This, in turn, sets up a situation where erosion can occur, further reducing soil organic matter. Without the store of carbon for soil life, we see decreased plant growth, and without adequate plant growth, soil biology is compromised, causing a reduction in yield. Finally, we are left with a soil that has a reduced ability to sustain production.
If the soil degradation spiral starts with human activity and ends in depleted resources, the path to regeneration should start with the soil and work back. Adopting management practices that foster biological activity kick-starts the soil biology to decompose, sequester, and utilize soil carbon, which increases nutrient cycling. More nutrients and a better soil environment lead to improved soil structure, with better water-holding capacity making water and nutrients available when needed, especially during drought. Practices that enhance biological activity result in sustained pasture yields while restoring soil quality.
Strategies for Building Healthy Soils
Let’s consider the agricultural practices that help build healthy soil. In essence, we want to increase aggregation, contribute soil organic matter, increase biodiversity, buffer soil temperature, and minimize soil compaction and disturbance. Sounds like a lot, right?
Well, not really, if we break them down into six basic principles. Let’s take a quick look at the principles that will define our soil management practices:
- Minimizing tillage preserves soil structure, encourages aggregation, and keeps soil carbon in the soil profile where it belongs. Tillage brings a flush of oxygen into the soil that spurs microbes into a feeding frenzy on carbon molecules… resulting in CO2 release. We reduce tillage through the use of perennial pasture and minimum or no-till of cover crops.
- Maintaining living roots in the soil for as much of the year as possible feeds soil microorganisms all year.
- Also, by maintaining living roots and leaving grazing residual, we are covering the soil all year, forming an “armor” to protect it from loss of moisture and nutrients.
- Maintaining species diversity is achieved with cover crop mixes and the use of diverse perennial-pasture mixes. Try to incorporate warm-season and cool-season plants, both grasses and broadleaf plants, in the same fields.
- Managing grazing is accomplished by planning for an appropriate grazing-recovery period on your paddocks, keeping in mind that plants need various recovery periods depending on the species, the time of year, and the soil moisture content. Overgrazing (not allowing adequate recovery) reduces root mass, photosynthesis, and the amount of carbon sequestered into the soil, decreasing soil life. Proper grazing builds soil.
- Finally, utilizing animal impact and grazing impact provides nutrient cycling in pastures, and contributes to soil organic matter. Additionally, the grazing action on forage plants encourages root growth and root exudation of plant sugars that feed soil microorganisms.
For livestock producers, this boils down to a combination of perennial pasture, cover crops in rotation on annual fields, and good grazing management. These simple concepts are described by ranchers Allen Williams, Gabe Brown, and Neil Dennis in a short video on how grazing management and cover crops can regenerate soils. View the video Soil Carbon Cowboys to get their take on soil health practices.
Grazing
Grazing Dynamics
Perennial pastures, because of the lack of soil disturbance and their permanent cover, are higher in carbon and organic matter than tilled crop fields. This biological system has a stable habitat to conduct business, and the nutrient cycles can sustain themselves. However, we know that by adding livestock to the mix, we get a multiplier effect on soil health.
The impact of grazing is known to increase soil carbon and nitrogen stocks. As an animal grazes, it sends a signal to the plant to pump out sugars through its roots and into the surrounding soil, or rhizosphere. Recall that root exudates, sugars developed by the plant through photosynthesis, are food sources for myriad microorganisms in the soil. The action of grazing jump-starts the soil food web and increases nutrient cycling, making nitrogen, phosphorus, and carbon available to the growing plants.
Walt Davis, a rancher and management consultant, puts it this way: “Grazed forage pumps root exudates (mostly carbohydrates) out into the area around their feeder roots; this feast of sugars and starches brings about an explosion of bacteria which causes the populations of predator microbes to increase greatly. As the predators consume the bacteria they take in more protein than they need and excrete the excess nitrogen (in a plant usable form) out right where the plant that contributed the carbohydrates can grab it to produce new growth. All of this activity (the life and death of billions of organisms) plus the root growth of properly grazed forage swards creates the fertile and biological active top soil that is the real solution to sustainable, productive and profitable agriculture” (Davis, 2017).
Cover Crops and Grazing
Cover crops are known to benefit the soil by feeding soil life, buffering temperatures, and increasing water-use efficiency. Many crop farmers are familiar with cover crops, but with livestock and cover crops in combination, you’ve got all the tools you need to build soil health. Grazing is often the missing link for crop farmers. By putting animals on cover crops, you can close the loop and develop a more resilient agricultural system.
Think of livestock as biological “roller-crimpers,” or cover crop terminators. The below-ground effects of grazing on root exudates, combined with the biological contribution from animals, far exceeds the benefits of cover crops alone. Because the microbes in the animal’s rumen are similar to the microbes in the soil, animals prime the soil with biological life, contributing to the health of the soil.
Cover crops not only provide excellent food for soil microorganisms but are high-quality forages for grazing livestock. Diverse cover crop mixes can provide excellent gains and milk production. Think of small grains such as wheat, rye, triticale, oats, and barley. Another good annual grass is annual ryegrass, which germinates easily, provides lots of biomass, and is especially good in a mix with clovers. Adding crimson clover, arrowleaf clover, berseem clover, sweet clover, hairy vetch, birdsfoot trefoil, or field peas provides livestock with protein and contributes nitrogen to the soil system. Most researchers and graziers suggest at least a 30% or greater composition of legumes in pastures for nitrogen-fixation benefits.
For a multi-year treatment, say three years or more, alfalfa works well. It’s deep-rooted and persistent for several years. Other cover crop species to consider are phacelia, mustard, turnip, kale, radish, rape, turnips, and yellow mustard. These are scavengers of nitrogen, and Daikon radish is a good subsoiler.
For summer, consider forage soybean, sorghum sudan, buckwheat, velvet bean (which is especially good with sorghum sudan), pearl millet, chicory, and sunflower. There are so many species to mix to keep pastures diverse and covered year-round while providing good grazing forages. SARE’s publication Managing Cover Crops Profitably has some good information on selecting cover crop species and mixes.
If you’re a farmer who has a predominately cash-crop-oriented income, it may be attractive to graze rotated cover crops as well as produce cash crops. Annual crops can be rotated to perennial pasture every few years. You can also incorporate grazing of cover crops in a strictly cash-crop system, as Gabe Brown has demonstrated. His fall biennial crop –> warm season cover crop –> fall biennial crop –> cash crop rotation works well in his system. Here you only have one year off from cash crop, but you get three cover crops in the crop rotation, with all of them grazed. This cover crop sequence works very well to “prime” depleted soils.
Take a look at Gabe Brown’s talk on cover crops.
How to Manage Grazing in Two Easy Steps
There’s a lot of information available about rotational grazing, multi-paddock grazing, and controlled grazing… it has a lot of names. As well, many graziers will extol the benefits of rotating animals from paddock to paddock. Paddock rotation is a management practice that helps us control grazing and has been shown to increase the sustainability of grazing operations. A system that rotates livestock through paddocks can be reduced to two essential steps:
First, determine how long your rest, or plant recovery period, should be. Then, determine how long animals should be on a paddock. Everything else in paddock rotations stems from these two decisions.
Recovery Period
Recovery period is the number of days that animals are not on a paddock, when the forage has a chance to recover from grazing. A rule of thumb is to start the spring with fast rotations, say moving cycling livestock every 15 to 20 days and increasing the recovery period as it gets warmer and dryer… to 30 days or so for cool-season grasses and 40 or more for warm-season grasses. These figures are for improved pasture in the Northeast, so a bit of judgement and adaptation for your region is crucial here.
Grazing Period
Once you know your recovery period, determine how long animals will be on the paddock. The animals should be removed from a paddock before grazed forages begin to regrow, to prevent overgrazing. For most forages in rain-fed pastures, this is around two to three days. Grazing periods of a day or less, especially with high animal density, provide uniform grazing and efficient manure distribution. High stock density builds soil. More about that later.
It’s important to maintain an adequate post-grazing residual forage height for photosynthesis and the recovery of carbon and nitrogen stocks. Remember that severe defoliation decreases carbon and nitrogen over time. A good grazing policy is to take half and leave half. This feeds both our livestock and soil microbes, resulting in less added fertilizers.
Remember: Managed grazing with adequate residual heights encourages soil aggregation, which is compromised with poorly stocked grazing. Situations that result in compaction should be avoided, such as grazing wet soils. Paddock grazing periods that are too long result in overgrazing. This is especially detrimental when the grazed paddock is not allowed full recovery before re-grazing.
For more on grazing recovery period, grazing period, scheduling, and setting up paddocks, see the ATTRA Grazing Planning Manual and Workbook. This manual is a workbook for writing your own grazing plan. It starts with a discussion of grazing principles and how to conduct a resource assessment, followed by a step-by-step process for writing a grazing plan.
As mentioned before, livestock grazing contributes to nutrient cycling and increases soil organic matter. Managed grazing allows for uniformity of paddock defoliation but also encourages uniformity of manure distribution within the paddock. The spatial distribution of manure can be managed in many ways: for instance by the size and shape of the paddock, the placement of water sources, and the number of animals on the paddock.
Paddock size should be determined by an assessment of the forage demand of the livestock and the forage available in the pasture. This will also determine the number of paddocks you need in a grazing cell. Lesson 3 of the ATTRA Managed Grazing tutorial covers this concept, and I recommend reviewing the principles to determine paddock size and number. The ATTRA Grazing Planning Manual and Workbook provides worksheets and a calculator to help you determine paddock size and number.
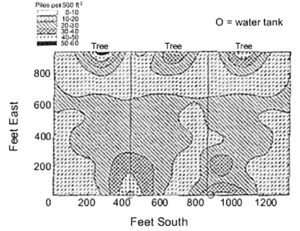
Figure 5. Manure distribution in a 3-paddock system. Source: NCAT
Square paddocks work best for encouraging uniform grazing and, thus, uniform manure distribution. Some practitioners suggest that long, narrow paddocks don’t work very well because livestock will graze one end of the paddock more intensely than the other. However, some have noted that with high stock density, livestock will graze a rectangle-shaped (or really any other shape) paddock just fine… working from one end to the other. The key here is animal numbers, as well as the length of time they are on the paddock. Long, rectangular paddocks can work if animal numbers are high and the grazing period is very short, creating a very high stocking density.
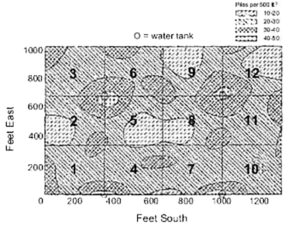
Figure 6. Manure distribution in a 12-paddock system. Source: NCAT
Another way to encourage even grazing distribution is with water-source placement. Water should be placed within 300 feet of the farthest reaches of the paddock for best distribution, but still, manure tends to accumulate toward the water source, especially as the temperature increases. Research has shown that, during heat stress, most dung accumulates within 100 feet of the water source. Shade and extra water sources can help alleviate this problem.
Let’s take a look at three different paddock systems and the influence that number of paddocks has on manure distribution. In the three-paddock system in Figure 5, it’s easy to see that the cattle congregate around the trees and the water source. Most likely, this system has long grazing periods, which increases grazing selectivity. It also clearly decreases uniformity of grazing.
The 12-paddock system in Figure 6 is a little better. Notice that a higher number of manure piles is distributed around the paddocks.
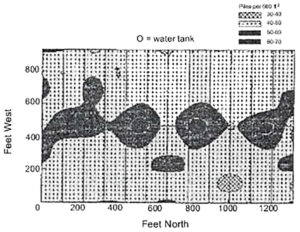
Figure 7. Manure distribution in a 24-paddock system. Source: NCAT
Finally, in the 24- paddock system in Figure 7, we see even more piles distributed throughout the paddock, even if there tends to be a high amount around watering sources. Compare this system with the three-paddock system. The more paddocks you have, the better the distribution of manure over the whole pasture.
Water tank placement, short grazing periods with high animal density, and more paddocks can maximize grazing distribution. In a well-designed pasture system, like the 24-paddock system, animals will deposit about 85% of their manure and urine within the paddocks, about 12% around water sources, and the rest in the lanes and, for dairies, the parlor.
So far we’ve discussed the soil environment, the soil life, the processes that occur through the work of microorganisms to make soil fertile and structurally sound, and some strategies based on soil principles that build healthy soil… notably the use of perennial pasture, cover crops, and good grazing management. Now, we will take the next step to identify how to make the transition to sustainable, resilient pasture soil fertility.
Making the Transition to Sustainable Pasture Fertility
It seems like there is a lot involved in managing pasture fertility holistically… and there is. The biological processes are complex and they inter-relate with weather, moisture, season, crop selection, livestock, and wildlife. Even soil scientists don’t understand everything that goes on in the soil, but we do have a pretty good idea of the processes and we know that biology is the basis for soil function. And we know that energy drives the whole system.
Energy from the sun becomes plant sugars and is primarily pumped out through plant roots to feed a diverse population of microorganisms, and secondarily used to fuel plant growth above ground. With the concept of energy transfer in mind, we can offer some steps in making a transition to ecological pasture fertility.
Transitioning to a biological system from a chemical system is going to be a slow process, and it’s good to recognize that it will take several years for soils to start turning around. Be patient and, as Ray Archuleta, a soils conservationist with NRCS, says, “you cannot build ecology integrity without human integrity” (2017). Have the integrity to believe that nature will work with you over time; that it’s going to work.
This is important, because there are going to be some problems that crop up as you progress. It could be anything from decreased weaning weights on calves, to weed problems, to livestock parasites. Expect these problems to occur, because you’re dealing with a biological system that is trying to get back into balance. Don’t jump ship at the first obstacle and succumb to the temptation to revert to an input-based system. Quick fixes don’t always work here. Resilience and the integrity to stay focused will pay off in the years to come as the biology builds to the point of sustainability.
Managing the biology to improve and maintain agricultural production requires adaptive management and keen observation… and the willingness to try new things. Put your ideas to work on whatever scale you can, and notice the changes. Keep what works and discard what doesn’t. Be your own research farm. Learn to solve your problems with biology rather than treating the symptoms with inputs… whether those are fertilizers or pesticides. This is the integrity Ray was speaking of. The long-term benefits are worth it, and it’s being done by farmers and graziers across the country.
Manage the Soil Carbon Liquid Pathway
So, how do you get started? Remember the three practices we spoke of earlier: perennial pasture, cover crops in rotation, and good grazing management. These practices build soil carbon, which is the key to fostering soil health and plant fertility. Christine Jones points out that there are four principles on which farmers can base their practices to enhance the liquid carbon pathway (2014):
- The first is to provide year-round living cover: for example, perennial pasture or annual cash crops/annual cover crops, so there are actively growing roots in the soil for as much of the year as possible.
- Next, enhance the soil’s biological activity by reducing nitrogen and phosphorus fertilizers that disrupt the flow of carbon from microbes to plants. Christine Jones suggests a 20% reduction in the first year, a 30% reduction in the second and third years, and thereafter small amounts of nitrogen, perhaps five to 10 pounds per acre per year, to kick-start the soil life in the spring.
- Then, promote plant and microbe diversity by incorporating short, medium, and tall-statured plants and a variation of cool-season and warm-season grasses and broadleaves for cover crops, as well as for perennial pasture mixes.
- Finally, use high stock-density animal impact for stimulating the activity that grazing has on plant roots and associated processes and microorganisms; namely nutrient cycling, water retention, and the production of humus. Graze grasses when they’re taller to maximize carbon sequestration. This helps build soil carbon and sets the stage for building healthy soil. Maintain animal production with high-density, short-duration grazing, giving the livestock the opportunity to be nonselective in their grazing, yet stimulate ample forage consumption. And when grazing, make sure to take half and leave half, to maximize nutrient cycling and carbon loading by trampling 1,500 to 2,500 pounds of dry matter per acre into the soil.
Making the transition takes time and attention, but the benefits are long term. Think of it as an investment in your soil, just like you invest in livestock, equipment, and crop seed. And as you begin this journey of renewal, remember that it’s a biological system that’s fully dependent on the almost incomprehensible diversity of life and life processes that happen unseen, among the roots just under the soil surface.
Compost and Compost Tea’s Role in the Transition
Compost can jump-start your transition to biological farming. Compost and compost tea supply a mix of soil microorganisms and the carbon for them to feed on. Make sure your compost is actively aerated and contains the proportions of bacteria, fungi, protozoa, and nematodes appropriate to your soil’s needs. Earthfort’s Soil Food Web analysis is necessary to do this. Try to apply a sufficient amount of compost to each acre every three years. You will notice the difference within a year in fields where compost has been applied.
Keeping It Simple…
Keep it simple and make a start, perhaps with one practice, like planting a cover crop on a small parcel, and build on it. Remember, your soil needs adequate organic matter to be able to cycle nutrients… upward of 4%. Closely monitor your animal and forage production. Try to look at things in a holistic manner and to resolve problems by observing how nature works and trying to mimic her. And, as stressed before, expect unforeseen outcomes to arise… it is a biological system you are farming.
And, importantly, have a financial reserve to see you through the first few years. This will help your cash flow during the transition.
It took decades for your soil to be degenerated, so expect several years for your farm to transition. Don’t make the mistake of expecting to reverse the tide in one year. And, as you transition, keep in mind the following concepts that have been covered in this publication:
- When you feed the soil microbes, you feed the plant… productivity is based on the relationships between plants, soil, and animals.
- The soil carbon pathway is the mechanism for nutrient transfer, and the process is kept strong by adding organic matter.
- Reduce your off-farm inputs to reduce cost.
- Transition slowly and have integrity in believing that it will work by staying the course even when the system seems to crash. Observe and adapt.
- If your soil is low in carbon, don’t expect the system to work. To fix it, start by putting in one or two years of cover crops and graze it appropriately to get the system primed. You might be surprised by the results in five years.
This publication has covered very little about nitrogen, phosphorus, and potassium… as well as pH, calcium, and all the nutrients and soil conditions that plants need to be productive. After having made it this far you can see why. We are talking about a biological system that, if healthy, provides nutrients to plants when they need them, from the workings of the liquid carbon pathway fueled by the sun working on organic matter through the facilitation of soil organisms.
To sum up, the physical attributes of soils include aggregation and all the benefits of good soil structure; the chemical attributes allow for nutrient cycling and the carbon pool; and the biological attributes are the dynamo that creates soil function. Maintaining the liquid carbon pathway in the soil is the key to fostering soil health and plant fertility. Managing for carbon by keeping soils covered with green, growing plants— and with managed defoliation through grazing— builds the organic matter that provides the fertility pastures need to be productive. Farmers can then rely on the soil microbes to cycle nitrogen, phosphorus, potassium, sulfur, water, and other nutrients to their pasture forage species. Feed the soil microbes, feed the crop.
Soil carbon is the substrate that provides energy for the soil microorganisms that drive the soil food web… the system of interactions between plants, roots, fungi, bacteria, and protozoa that supports all life above and below the soil surface. Nitrogen fertilizers are known to inhibit the exudation of sugars that feed the microorganisms that form soil humus, a stable form of soil carbon. Therefore nitrogen management is crucial for the soil life that supports crop production.
Farmers are aware of the nitrogen-fixing capabilities of rhizobium bacteria, the microorganisms that, in symbiosis with legumes, fix atmospheric nitrogen. But in addition to plant-associated nitrogen fixation, healthy soil is full of bacteria that fix nitrogen without direct plant associations. Non-legume nitrogen fixation is accomplished by soil bacteria and archaea. It is now known that the more growing plant cover (think more photosynthesis) there is on the soil surface, the more of these microbes there are, doing their work, beneath the soil.
Connecting everything together and forming the pathways that facilitate nutrient transfer are the mycorrhizal fungi. These fungi soak up carbon in the form of sugars from plant root exudates and deliver nitrogen and other nutrients to plants throughout a web of hyphae that extend well past the individual roots of the plants. These fungal hyphae yield the glues that bind soil together into aggregates that give healthy soil all the characteristics we look for, including water holding capacity, soil tilth, nitrogen fixation, and carbon sequestration.
Building Healthy Pasture Soils
By Lee Rinehart, NCAT Agriculture Specialist
Published October 2017
© NCAT
IP546
This publication is produced by the National Center for Appropriate Technology through the ATTRA Sustainable Agriculture program, under a cooperative agreement with USDA Rural Development.
Related Publications
- Managed Grazing Tutorial
- Nutrient Cycling in Pastures
- Cover Crop Options for Hot and Humid Areas
- Integrating Livestock and Crops: Improving Soil, Solving Problems, Increasing Income
- Overview of Cover Crops and Green Manure
- Tipsheet: Assessing the Soil Resource for Beginning Organic Farmers
- Pasture, Rangeland, and Adaptive Management
- Irrigated Pastures: Setting Up an Intensive Grazing System That Works