Genetically Modified Crops: Transgenics and Cisgenics
By Nancy Matheson and Katherine Adam, NCAT Agriculture Specialists; and Jeff Schahczenski, NCAT Agricultural and Natural Resource Economist
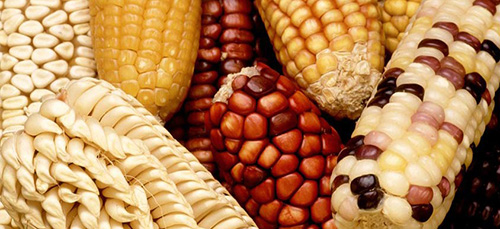
To increase the genetic diversity of U.S. corn, the Germplasm Enhancement for Maize (GEM) project seeks to combine exotic germplasm, such as this unusually colored and shaped maize from Latin America, with domestic corn lines. Photo: Keith Weller, USDA ARS
Abstract
This publication analyzes the utilization of transgenic and cisgenic crops in agricultural production and the consequences they create for farmers, ecosystems, and the economy. It provides an overview of the main agricultural crops that have been genetically modified, the characteristics they express, and the market roles they play. It also discusses the potential unintended consequences of using transgenic and cisgenic GMOs in agriculture. It also addresses seed, and agrichemical market concentration and raises human and environmental safety concerns regarding the cultivation and dissemination of genetically modified crops. In addition, the publication addresses economic, legal, and management concerns associated with these types of crops, as well as policy and regulatory aspects. Lastly, the publication explores implications of genetic modification technologies for sustainable agriculture. References and resources follow the narrative.
Introduction
Genetically modified crops and potential consequences following their utilization in agricultural crop production are the subject of this publication. There are two types of genetically modified crops that have been utilized and regulated in U.S. agricultural production—transgenic and cisgenic crops. Transgenic crops have had genetic material transferred between two unlike species. Cisgenic crops have genes that have been “edited” within that species. The term “gene editing” is used widely in the general public literature.
Scientific development in the methods of producing genetically modified crops began around 1983 as part of a broader technological movement to genetically modify organisms for economic, medical, military, and other general human ends (transgenics). Cisgenics, a more recent form of genetic modification, began around 2015. Both methods of genetic modification have positive and negative (often unintended) consequences when used in agricultural production. Cisgenics are not yet subject to the same level of regulation as transgenic crops.
The following data reveal how transgenic crops have been increasingly utilized and regulated in U.S. agricultural production. Corn, soybean, and upland cotton are the major U.S. transgenic crops tracked by USDA. As of 2017, the National Agriculture Statistics Service (USDA/NASS) reported that transgenic varieties comprised 84% of all soybean acreage planted in the United States (up from 60% in 2001). As of the same date, transgenic corn acreage planted was 92% (up from 47% in 2004). Transgenic upland cotton was 96% (up from 76% in 2004).
No acreage report was provided for transgenic varieties of other U.S. crops, such as sugar beets, canola, alfalfa, and apples (USDA/NASS, 2017). For information on additional genetically modified crops that are approved, see the International Service for the Acquisition of Agri-biotech Applications database (ISAAA Database).
What are Genetically Modified Crops?
Biotechnology: Transgenic and Cisgenic Crops
No uniformly accepted definition of biotechnology exists, according to the National Agricultural Law Center’s Biotechnology website. The Center provides several definitions and commentary:
- “Under the broadest definition, the use of biological sciences to develop products, conventional plant and animal breeding techniques, conducted since the dawn of civilization, fall under biotechnology. In the popular press, biotechnology generally refers to newly-developed scientific methods used to create products by altering the genetic makeup of organisms and producing unique individuals or traits that are not easily obtained through conventional breeding techniques. These products are often referred to as transgenic, bioengineered, or genetically modified because they contain foreign genetic material.” (National Agricultural Law Center, 2018)
New genetic engineering methods, broadly referred to as “gene editing,” also known as “New Genetic Modification Techniques” (NGMTs), have been increasingly utilized (ENSSER, 2017). Gene editing represents newer methods of genome manipulation, referred to as cisgenics (Fess and Benedito, 2018). Agriculture is one of the first industries significantly affected by cisgenics on fundamental production, economic, political, and legal levels (National Agricultural Law Center, 2018).
Transgenics, the genetic modification process utilized in U.S. agriculture many years before cisgenics, refers to methods by which biologists splice genes from one or more unrelated or distantly related species into the DNA of crop plants in an attempt to transfer chosen genetic traits (this method is also known as “recombinant DNA technology”).
Genes are segments of DNA containing information that, in part, determines the end function of a living organism. Transgenic engineers manipulate DNA by taking genes from one species—an animal, plant, bacterium, or virus—and inserting them into another species, such as an agricultural crop plant. The desired transgenes are delivered in a “package,” of exogenous nucleic acids (DNA from other species) that includes a marker gene—typically coding for resistance to a specific antibiotic—to verify the intended result of the gene transfer. “Gene stacking” means the package delivers an array of several traits at once into the host organism.
Often, an intermediate organism or virus can be used to “infect” the host DNA with the desired genetic material. Another technique is micro-particle bombardment, in which the desired genetic material is precipitated onto micron-sized metal particles and placed within one of a variety of devices designed to accelerate these “micro-carriers” to velocities required to penetrate the plant cell wall and membrane. In this manner, transgenes can be delivered into the cell’s genome. Electroporation allows DNA to be inserted into a host cell when a jolt of electricity is applied to create openings in the plasma membrane that surrounds a cell.
Cisgenics (gene editing or NGMT) involves a set of new techniques that are not transgenic and therefore do not introduce genes from another species but rather manipulate the genome of a single plant or animal species. Sometimes, gene editing introduces human-made “synthesized” or “manufactured” genes into the host cell.
There are three common types of cisgenic techniques utilized in crop modification: CRISPR (Clustered Regularly Interspaced Short Palindromic Repeats), TALEN (Transcription activator-like effector nucleases), and RNAi (Ribo-nucleic acid interference). So far, these techniques are not significantly regulated in the United States because current regulations of modified crops have been specific to transgenic modification.
The transfer of desirable genetic traits across species barriers or within a specific plant offers potential promise to solve problems in the management of agricultural crops and provide new possibilities to improve human and animal health—and may also provide new revenue streams for farmers through contract production of pharmaceutical and industrial crops.
Potential environmental benefits claimed by genetic modification are reduced toxic-pesticide use, improved weed control resulting in less tillage and soil erosion, and potential water- and nutrient-use efficiency. Furthermore, the new technology sometimes promises increased yields. Gene-edited techniques open the possibility of designer crops and vegetables, adding, for example, flavor and nutritional values. For instance, one of the first examples of a cisgenic crop to be commercially available will be a soybean that produces oil that is more nutritionally balanced and closer to olive oil (MIT Technology Review, 2018).
It is important to note that transgenic crops are also patentable. Technology agreements ensure that transgenic seed cannot be saved by the farmer for planting the next year. The developer’s intellectual property rights are thereby protected, which offers the potential to increase profits and often creates a near monopoly over the genetically modified seed supply of a particular crop.
Unintended Effects and Consequences of Utilizing GMO Crops: Pleiotropy
Transgenic and cisgenic methods of gene-transfer and manipulation are not precise. Although scientists can control—with relative exactness—the trait gene (or its synthesized analog) to be inserted into a host plant genome, they cannot entirely control its location, nor the number of copies that get inserted.
Location of genetic material is important because it controls the expression of biological traits, just as genes themselves do. Also, inserted DNA frequently contains multiple stacked genes for different traits (eight in the genetically modified New Leaf potato), increasing chances of undesirable or unknown interactions.
A common and unpredictable occurrence in transgenics is “silencing” of either the inserted genetic material or adjacent native genes. Present scientific knowledge is still a long way from being able to control precisely the traits the host plant will express and guaranteeing genetic stability in subsequent generations (Ryan and Ho, 2001).
This potential for instability through silencing can lead to unpredictable and undesirable effects. Examples of this are plant infertility, production of toxins and allergens, and reductions in yield and plant fitness. The transgenic seed industry consistently counters that because genes from no known allergens are incorporated, adequate care has been taken to guard against this contingency (USDA/OIG, 2005). The problem is that there can be hundreds of unintended mutations from transgenic and gene editing manipulations, and it is simply impossible to know easily what human-health or environmental impacts may result. These mutations are referred to as “off-target,” and there is great controversy about possible impacts of these mutations in both agriculture and medical uses of genetic modification (Schaefer et al., 2017; Latham and Wilson, 2015).
Pleiotropy is the concept that one gene may control multiple traits in an organism. The model of gene expression is not simple. The idea that one gene equals one effect is simply false. This misunderstanding has had serious implications in a variety of contexts related to the regulation and utilization of genetically modified crops in agricultural production.
Pleiotropy multiplies the uncertainty surrounding the usage of transgenic crops. A single gene identified as controlling a desirable characteristic may, in fact, control multiple characteristics, and in a variety of ways. Pleiotropy is common, and the interactions of genes with each other and with the environment adds a significant level of complexity. Pleiotropy reveals that accurately predicting the effects of new genetic combinations is nearly impossible.
In addition, the introduction of a novel life form into an ecology can trigger effects perhaps too great to be understood during short periods of time. Although it is true that many mutations might not survive in an environment, those that do could profoundly affect other life forms, including humans.
Cisgenics only manipulates the genome within a specific plant; therefore, these techniques may have less of an issue with pleiotropy. Cisgenic crops appear to be more like the result of classical breeding in terms of expected impacts on food safety and environmental consequences.
There have been counter-claims that “unintended” changes in genomes can often occur when cisgenic techniques are used (ENSSER, 2017). Thus, significant uncertainty still exists regarding whether genetically modified crops may exhibit traits that could have negative consequences on human and environmental health.
Commercial Transgenic Crops and Their Traits
Increased yields, shelf-life, and improved nutritional value are among the promised benefits of genetically modified crops. For example, a transgenic tomato was designed for long shelf-life. Transgenic “Golden Rice” (and now “Golden Rice 2”) was designed to increase beta-carotene (Vitamin A) in a usable form for humans, though no commercial production has yet taken place (Hilbeck and Herren, 2016). There has been recent introduction of drought-resistant transgenic corn, but it is not yet clear that the yield benefits are significant compared to other, non-GMO varieties. Nonetheless, most of the genetically modified crops that are planted worldwide are designed with these aims in mind: 1) to survive exposure to certain herbicides (called herbicide tolerant, or HT); 2) to kill certain insect pests (called pesticidal or insecticidal); or 3) to be both herbicide tolerant and insecticidal (often called “stacked” traits).
Transgenic herbicide-tolerant (HT) crops have been altered to withstand being sprayed with broad-spectrum herbicides, with the idea that one application will take care of most types of weeds without killing the crop. Insecticidal crops contain genes of the soil bacterium Bacillus thuringiensis (Bt). These Bt genes cause the plants to produce a chemical toxic to the European corn borer, corn root worm, cotton boll-worm, and other caterpillars. Stacked transgenic crops have combined these two types of genetic modifications in a single crop.
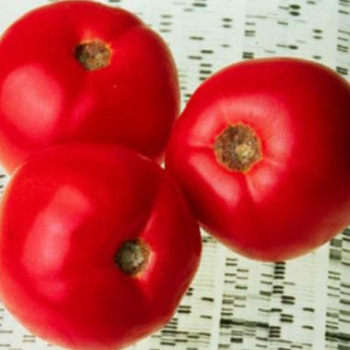
A retooled gene in Endless Summer tomatoes controls ripening to give better flavor and shelf-life. Photo by Jack Dykinga, USDA ARS
With an overwhelming amount of U.S. commodity crop (corn, soybean, upland cotton, sugar beets, and canola) acreages devoted to transgenic versions, seed for conventional (non-GMO) varieties is often scarce for those who choose not to plant transgenic crops. Soybeans and corn, often grown in rotation in the upper Midwest and other major commodity-crop-growing regions, account for the largest acreages in transgenic crops. Non-GMO seed scarcity can affect farmers who wish to maintain or return to non-transgenic crops (Holden, 2002). Development of the non-transgenic and organic/specialty cotton sector has been hampered by concerns about cross-pollination and boll-weevil control.
One other larger-acreage North American transgenic crop is canola (a low-erucic-acid form of European rapeseed). Canola is a major oilseed crop in Canada and an expanding crop in the United States. Canola production has increased in the Pacific Northwest and North Dakota; much of it is transgenic varieties (USDA/NASS, 2017). Smaller-acreage vegetable seed producers in the Willamette Valley of Oregon have raised concerns that transgenic canola could cross-pollinate with related crucifer-vegetable crops, thus threatening a valuable organic and non-GMO crop-seed industry.
Beginning in 2011, canola production in Oregon initially grew from 5,300 acres to 11,000 acres, then dropped to just 4,000 acres in 2016 (USDA/NASS, 2017), largely as a result of controversy over potential impacts of transgenic canola on vegetable-seed growers. In 2013, the Oregon State Legislature passed a law that prevents efforts by local governments to ban genetically modified crops, yet canola production in the Willamette Valley appears limited, at least until 2019, because of an earlier agreement.
Farmers’ Concerns: Transgenic Crops
Since 2001, ecological and human safety concerns of transgenic crops have become evident. These concerns include the following:
Gene Flow to Neighboring Crops and to Related Wild Species
Gene flow from transgenic fields into conventional crops and related wild plants is an issue of special concern to farmers because of the potential for crops to develop herbicide resistance. In western Canada, three different herbicide-resistant canola varieties have cross-pollinated to create canola plants that are resistant to all three types of herbicide. This new “triple resistance” has turned volunteer canola into a significant weed problem (Ellstrand, 2001).
Gene flow from transgenic crops to wild relatives causes wild plants to acquire traits that improve their “fitness,” turning them into “super weeds.” For example, jointed goat grass—a weedy relative of wheat—can acquire the herbicide-tolerant trait of a yet-to-be-released genetically modified wheat, and could therefore thrive in crop fields unless applications of other herbicides are made. Frank Young and his colleagues at Washington State University found that imidazolone-resistant wheat (a classically bred variety) outcrossed to goat grass in one season (Stierle, 2018). Other traits that wild plants could acquire from transgenic plants that will increase their “weediness,” are insect and virus resistance (Ervin et al., 2001). Alfalfa, a popular hay crop, can easily cross with black medic, an invasive species prevalent in the western United States.
Counter arguments from the transgenic crop industry claim that resistant weeds can be controlled by “other herbicides.” Researchers at Iowa State University’s Leopold Center found that the increased cost of using other herbicides negates any cost advantage to the farmer of using transgenic seed (Benbrook, 2001). In addition, recent efforts to address glyphosate resistance in weeds by developing additional transgenic cultivars resistant to dicamba have already had the unintended consequence of drift-related damage to non-target crops from this highly volatile herbicide. Furthermore, development of new transgenic crops resistant to additional herbicides will simply promote development of weeds resistant to these herbicides, resulting in a transgenic-herbicide treadmill (Bain et al., 2017). Because of potential effects on pest management, crop marketability, and liability, more research is needed to determine the conditions under which gene flow from transgenic plants is likely to be significant.
Pesticide Resistance in Insect Pests
Bt has been widely used as a microbial spray because it is toxic only to caterpillars. In fact, it is a pest-management tool on which organic farmers partially depend—one of the few insecticides acceptable under organic rules. Unlike the commercial insecticide spray, the Bt engineered into transgenic crop plants is reproduced in all, or nearly all, the cells of every plant, not just applied on the plant surface for a temporary toxic effect. As a result, the likelihood that transgenic Bt crops will accelerate development of pest resistance to Bt is of serious concern. Such resistance would remove this valuable and environmentally benign tool from the pest-control toolbox of organic farmers and forest managers.
In a recent article, authors reviewed data for 36 cases in 10 countries and found pest resistance that substantially reduced the usefulness of Bt crops in 16 cases in 2016, compared to only three such cases in 2005. However, in 17 other cases, no Bt resistance was found in 2016 and, in these cases, Bt remained effective after 20 years of use (Tabashnik and Carrière, 2017).
Effects on Beneficial Organisms
There are many instances in which transgenic and possibly cisgenic crops have and/or may have unintended effects on beneficial organisms. Studies show that Bt crops exude Bt in concentrations high enough to be toxic to some beneficial soil organisms. Use of glyphosate and the various formulations of glyphosate products also appear to impact soil organisms negatively (Soil Association, 2016). University of Arkansas agronomists found impaired “root development, nodulation, and nitrogen fixation” in herbicide-tolerant soybeans (King et al., 2001). Disruption of beneficial soil organisms can interfere with plant uptake of phosphorus, an essential plant nutrient (Massey, 2000).
Beneficial insects that prey on insect pests can be negatively affected by insecticidal crops in two ways. First, the Bt in transgenic insecticidal crops was shown in some laboratory studies to be toxic to ladybird beetles, lacewings, and monarch butterflies (Ervin et al., 2001). The extent to which these beneficial insects are affected in the field is a matter of continued study. Second, because the insecticidal properties of Bt crops function even in the absence of an economic threshold of pests, Bt crops potentially can reduce pest populations to the point that predator species are negatively affected.
The case of the decline of the Monarch butterfly is instructive. Although the initial research suggested that Bt crops were a potential source of that decline, later research revealed that the use of herbicide tolerant (HT) or “stacked” (mixed) HT and Bt transgenic crops led to the reduction of milkweed plants and the creation of “milk-weed deserts,” which implies severe decline in the breeding range of the Monarch (Bøhn and Lövei, 2017). Thus, beneficial organisms are both directly and indirectly impacted through herbicide-tolerant and Bt transgenic crops.
Antibiotic Resistance
The resistance of bacteria to antibiotics is a significant problem for human health. The transfer of genes for transgenic crops is accomplished by the use of what are called antibiotic-resistant marker genes. This practice carries the possible danger of spreading antibiotic-resistant bacteria into the environment (Breyer et al., 2014). Also, widespread planting of HT crops has logically resulted in increased use of herbicides to which the crops are tolerant. As weeds evolve resistance to these herbicides, farmers have been forced to increase their use of other types of herbicides.
Common herbicides used with transgenic crops include glyphosate, 2,4 D, and dicamba in various commercial formulations. This increased application of herbicides can also change the way bacteria respond to antibiotics. A recent study has found that the use of various common herbicides can cause antibiotic resistance at concentrations of the herbicides below the application rates recommended by manufacturers (Kurenbach et al., 2017). Additional research on this topic is required in order to assess regulatory considerations.
Genetic Diversity Reduction in GMO Crops
As fewer and larger firms dominate the customer base of seed and biotechnology markets, transgenic and gene-edited crops may continue the current trend toward simplification of cropping systems by reducing the diversity of crops and cultivars planted. In addition, seed-saving, which promotes genetic diversity, is discouraged and, in the case of patented cultivars, legally prohibited.
In Europe, seed-saving traditionally practiced by a majority of farmers has been heavily restricted through registration requirements and subsidy payments. To be certified, seed must exhibit “distinctiveness, uniformity, and stability,” called “DUS registration” (Toledo, 2002). A traditional landrace can be held uncertifiable (and effectively outlawed by billings for royalties and denial of subsidy payments) by being declared insufficiently distinct from a variety described in the EU Catalogue of Common Varieties.
Food Safety Concerns
Food-safety issues raised by transgenic crops are beyond the scope of this publication. However, possible food-safety concerns include the following:
- Possibility of toxins in food
- Possibility of new pathogens
- Reduced nutritional value
- Introduction of human allergens
- Transfer of antibiotic resistance to humans
- Unexpected immune-system and genetic effects from the introduction of novel compounds
These concerns are directly tied to the increased demand by domestic consumers for organically grown crops. Genetically modified foods will be legally required to be labeled/disclosed in the United States beginning in 2010.
Farm-Management Issues
The most widely planted transgenic crops on the market today can simplify short-term pest management for farmers. In the case of herbicide-tolerant crops, initially farmers hoped to use a single broad-spectrum herbicide for all their crop weeds. It has turned out that they need more than one application in most seasons and often require combinations of different herbicides to counter weed resistances. New genetically modified crops with tolerance to different herbicides (glyphosate to dicamba) have also been developed to counter weed resistance. By planting insecticidal crops, farmers can eliminate the need to apply pesticides for caterpillar pests like the European corn borer or the cotton bollworm, though they still have to contend with other crop pests.
Although these crops offer simplified pest/weed-control features, they may complicate other areas of farm management. Farmers who grow both transgenic, non-organic, and certified organic or certified non-GMO varieties of the same crop will need to segregate them during all production, harvesting, storage, and transportation phases if they sell into differentiated markets or plan to save their own seed from the non-GMO or organic crops. For an example, see the complete regulations for organic handling here. Whether the newest cisgenic crops will require segregation from non-organic, non-GMO crops is not yet known, but likely. Certified organic crops must be kept separate from cisgenic crops because these are still considered genetically modified, even if not transgenic.
To minimize the risk of gene flow from transgenic to adjacent non-GMO or organic crop fields, Federal regulations require buffer strips of non-GMO and organic varieties around transgenic fields. Different transgenic crops require different buffer widths. Because the buffer strips must be managed organically or conventionally, producers have to be willing to maintain two different farming systems on their transgenic and non-transgenic fields. Crops harvested from the buffer strips must be handled and marketed as though they are transgenic. Planted refuges—where pest species can live outside fields of insecticidal transgenic crops—are also required to slow the development of insect-pest resistance to Bt and broad-spectrum herbicides, respectively. These refuges allow some individuals in the pest population to survive and carry on the traits of pesticide and herbicide susceptibility. Requirements governing the size of refuges differ according to the type of transgenic crop grown.
Farmers growing herbicide-tolerant crops need to be aware that volunteer crops the following year will be still be herbicide resistant. Such resistance makes no-till or direct-seed systems difficult because volunteers can’t be controlled with the same herbicide used on the rest of the crop. In a no-till system that relies on the same broad-spectrum herbicide that the volunteer plants are resistant to, these plants will contaminate the harvest of a following conventional variety of the same crop—a situation farmers tend to avoid for two reasons. First, the contamination means a following crop will have to be sold on the transgenic market. If farmers grow and market a transgenic crop for which they do not have a technology agreement and did not pay royalty fees, they may face aggressive collection by the company that owns the transgenic variety. Hundreds of U.S. farmers have been charged with “theft” of a company’s patented seed as a result of contamination in the field (Altieri, 2000).
Farmers growing insecticidal crops need to recognize that insect pressure is difficult to predict and may not warrant the planting of an insecticidal variety every year. In a year when pest pressure is low, the transgenic seed becomes expensive insurance against the threat of insect damage (Hillyer, 1999), as well as unnecessarily increasing selection pressure for resistant pests.
Farmers growing transgenic crops need to communicate with their neighbors to avoid contaminating neighboring fields and to ensure that buffers are adequate. In Maine, farmers growing transgenic crops are required by law to be listed with the state agriculture department, to help identify possible sources of cross-contamination when it occurs. The law also “requires manufacturers or seed dealers of genetically engineered plants, plant parts, or seeds to provide written instructions to all growers on how to plant, grow, and harvest the crops to minimize potential cross-contamination of non-genetically engineered crops or wild plant populations” (AgBioTech InfoNet, 2001).
Crop Yield, Costs, Economics, and Other Impacts
Some farmers will get higher yields with a particular transgenic crop variety than with their non-transgenic varieties, and some will get lower yields. Yield variability is related to many factors, including choice of the non-transgenic analog of the transgenic variety, making it very difficult to analyze how any one feature impacts yield. Costs of various transgenic crop inputs (herbicides and seed, including associated “technology fees” charged by the patent holder) are also constantly changing; and the ability of farmers to adjust to changing costs—particularly rapid changes—is limited and affects profitability of both transgenic and cisgenic crops.
However, some yield, cost, and economic-impact trends do appear to be emerging from the growing body of research data on transgenic crops. (No published research is available on gene-edited crops, as they have only barely entered commercial markets as of this writing.) Two recent research efforts are instructive on these issues.
Case Study: Transgenic Corn Crops, Yields, and Lowering Crop Insurance Costs
Between 2008 and 2011, the USDA Risk Management Agency (RMA), which implements all Federal crop insurance programs, offered a reduced premium for insuring corn in a select group of states primarily in the Midwest, if farmers adopted multiple-gene-stacked transgenic seeds in the production of corn. This benefit was provided under a special RMA program that allows private entities (in this case transgenic corn seed producers) to develop special pilot insurance products.*
Figure 1. Yield Distributions for Conventional (NT) and Biotech (BT) Corn. Source: Goodwin and Piggott, 2018
The pilot was based on data provided by the transgenic seed companies (Monsanto, Syngenta, and Dupont) that documented a higher probability that the yield of the transgenic (bio-engineered) corn was consistently less variable than non-transgenic corn (Goodwin and Piggott, 2018). Hence, the transgenic corn was deemed less risk to grow and warranted a lower premium cost.
However, the evidence provided by the transgenic seed companies was in contradiction to much of the then-published research, which suggested the opposite result. That early and more current research, with some exceptions, suggest that although average corn yields for transgenic corn are likely higher, the yield “risk,” measured as the volatility of corn yields when exposed to extreme weather, is greater. In general, corn appears to be becoming more “sensitive” to environmental stresses. Also, other factors, particularly the rapidly changing density of corn planting, also appear to impact yield risks.
Interestingly, the pilot ended in 2011 because the argument was made that almost all corn was transgenic by then, and that it was better to set corn premium rates lower in general rather than have a special pilot program. However, a recent (2018) study for which the authors were able to obtain data on those participating in the pilot suggested that, at least when comparing the corn-yield impacts of major droughts in 2008 and 2012, transgenic corn appeared to be less risky to grow than non-GMO corn (Goodwin and Piggott, 2018). Thus, the yield benefits of transgenic corn seem to still be an unresolved issue needing further research.
*These pilot programs are approved by the Federal Crop Insurance Corporation (FCIC) under what is known as the 508(h) program, based on the statute section of the law that created the program.
General Economic and Other Impacts of Transgenic Crops
Some researchers took a unique approach to determining the economic impact of transgenic crops by asking the question: what if non-GMO were substituted for GMO crops in the United States? (Taheripour et al., 2016). More specifically, what would the economic, land-use, and greenhouse-gas (GHG) emission impacts be for such a change? The methodology used to answer these questions critically hinges on estimations of yield differences between GMO and non-GMO crops. A 2016 study examines three crops: corn, soybeans, and cotton.
The yield estimates in this study are made based on selective published research on yield differences between GMO and non-GMO corn, soy-beans, and cotton in the United States. Because the published research on estimated yield differences is not specific, but offers a range of yield differences, the researchers identify a reference yield (an upper-bound estimate), a conservative yield (a lower-bound estimate), and a simple average of the two estimates for each crop. The average estimate for each crop is given as the expected percentage decrease in yield if a shift were made from the GMO crop to the non-GMO crop. The average percentage yield decreases were estimated to be 11.2% for corn, 18.6% for cotton, and 5.2% for soybeans. The authors refer to these yield changes as yield or supply “shocks.”
Once these yield differences were estimated, the authors used this and other data to attempt to explore the national aggregate economic, land-use change, and GHG-emission impacts that such yield losses would imply by using an econometric computable general equilibrium model.* Various scenarios are created, called the base case (no adjustments to model), fixed-trade case (corn, soybean, and cotton exports remain constant), fixed-food case (food consumption held constant), and a combination case of the fixed-trade and fixed-food cases. The research results show “significant” impacts on land use and GHG emissions, as well as increases in commodity and food prices from eliminating GMO corn, soybean, and corn.
The significance of these impacts does vary considerably, depending on the scenario used and the underlying, reference, conservative, and average yields assumed. For example, the estimated changes in the supply price of corn when moving from GMO to non-GMO production under the base-case scenario with an average yield shock is an increase in price of 9.8%. However, for the same base-case scenario with the reference and conservative yield shocks, there are estimated price increases for corn of 17.1% and 3.8% respectively. Thus, the range of supply-price impacts varies highly, depending on yield and scenario assumptions. Similarly wide ranges of price impacts when shifting from GMO to non-GMO are also found for production, land-use changes, and GHG emissions.
Although this study does generally support a negative economic-welfare impact from banning the use of GMO corn, soybeans, and cotton, the measured impact is again highly variable with the reference, average, and conservative yield cases, resulting in an estimated economic loss of $1.1 billion, $0.6 billion, or $0.2 billion to the general U.S. economy.
Again, it is important to note that the outcomes of this assessment depend significantly on the yield estimates used. The authors used only a few studies to determine these estimates, and it is not clear that these are the only studies available. Also, in the case of corn and soybean yield estimates, the data come from a single USDA Economic Research Service (ERS) publication that averages farm-level data from a USDA Agricultural Resource Management Survey (ARMS) collected for a single year. Cotton yields are estimated from a single 2006 study for the reference case and a 2003 study for the conservative case. These studies have important limitations in that they are largely from older data and generally from a single year. All crop yields, whether transgenic or non-transgenic, can vary significantly across time. Indeed, a 2009 study by the Union of Concerned Scientists (UCS) concluded that GMO corn and soybeans did not provide improved yields compared to non-GMO corn and soybeans (UCS, 2009).
There are many factors that can impact crop yields, and it is not clear how these studies controlled for alternative factors that could have impacted yield differences. Further studies that look at yield differences between GMO and non-GMO crops are greatly needed to improve these types of general economic-impact studies of transgenic crops.
The 2016 study does not indicate the impact of using non-GMO or GMO crops on the profitability of an individual farmer. Indeed, given that almost all farmers in the United States have adopted transgenic corn, soybeans, and cotton, the claimed production costs and yield advantages of these transgenic crops have likely already been gained by the farmers using them. The initial potential lower costs and increased yield gains of GMO crops likely do not provide any further profit advantage that early adopters of this technology may have experienced. Indeed, the widespread adoption of transgenic crops has created new markets for non-GMO crops and has likely increased the demand for organic food products, to the extent that consumers remain leery of potential food-safety and health issues related to GMO crops, whether or not those concerns are warranted. In addition, the evolution of herbicide-resistant weeds and Bt-resistant pests in response to widespread implementation of these transgenic crop technologies has increased production costs and possibly reduced yield benefits.
* A general equilibrium model attempts to mimic a national or global economy. These models are based on many assumptions and are highly complex, and offer a macroeconomic view of given changes. How changes such as yield differences between GMO and non-GMO impact an individual farmer represent a microeconomic viewpoint that could be very different than aggregate impacts.
GMO Crops vs. Organic Crops
Another way to analyze the benefits and drawbacks of transgenic crops is to look at a comparison of certified organic crops with similar crops grown transgenically. A recent research paper undertook this analysis by posing a single question—a world without hunger: organic or GMO crops? (Taheri et al., 2017). The implication is that that the expected yield loss under organic production systems will ultimately result in world hunger.
This analysis is not based on specific farms and takes a global perspective. The authors note, for instance, that beginning in 2012, the acreage of transgenic crops in developing countries surpassed that in developed countries. They also warn against over-estimating the impact of transgenic crops on overall world crop production—because only about 10% of the world arable land is dedicated to GMO crops. Nonetheless, this study’s review of the research literature suggests the following positive outcomes from GMO crops:
- An estimated net economic benefit at the farm level of $17.7 billion globally.
- Economic gains are attributed to yield increases and lower-level costs of production.
- Estimated worldwide reduction in insect pesticide use by 37%.
On the negative side, the authors suggest the following:
- The technology has focused on labor and management savings and not on quality germplasm improvements.
- Positive yield and economic effects are not uniform and depend on varieties and location.
- Marketing GMO crops can cause difficulties because of continued consumer food-safety and health concerns.
- Generally, GMO crops negatively impact biodiversity.
When it comes to organic cropping systems, the authors also note potential positive and negative impacts. On the positive side, they note the following:
- The goal, if not the requirement, of organic production systems is to produce food with minimal harm to the ecosystem.
- Soil organic matter, and perhaps soil health, tend to be higher in organic production systems.
- Organic cropping systems may use less non-renewable energy.
- Organic cropping systems may be able to better handle production risks because of the need to grow a greater diversity of crops in rotations.
The authors note serious challenges to organic systems, as well:
- Learning how to grow organically is complicated and takes investment in time and resources to perfect.
- The three-year transition period to certified organic production is difficult economically because organic markets cannot be accessed during that time, and yields and income may decline during this period.
- Organic crop-production systems are knowledge intensive, and organic research into production and market issues is limited relative to GMO-crop-research funding.
The authors end their review by suggesting a co-existence between GMO and organic cropping systems. They also suggest that GMO commodity-crop production may be beneficial for developing countries, so as to provide greater cereal production for growing food demands. At the same time, they suggest that organic cropping systems may be better suited for Europe, the United States, and other developed-agriculture countries where issues of environmental consequences may take precedent over low-cost industrial production of commodity crops. However, other studies have also suggested that organic and other conservation agriculture systems can outperform higher-input industrial and transgenic-crop-based systems, even in developing countries (Ponisio and Ehrlich, 2016; UNCTAD-UNEP, 2008).
Impacts of GMOs on Organic Farmers
Organic farmers face even bigger marketing and trade risks from GMO crops because their buyers expect zero transgenic contamination. Currently, organic production is process-oriented, not testing-oriented—except for exports. The organic industry has a system for segregation, but tests for transgenic material in organic products demonstrate that organic agriculture is not immune to contamination from transgenic systems (Callahan, 2001).
New technologies can reliably detect minute amounts of transgenic material. Published reports from Europe and the United States confirm a high degree of accuracy for detection methods (Non-GMO Source, 2004). European export markets that U.S. organic farmers might have enjoyed, and those that producers of non-transgenic conventional crops could build upon, have proven unstable in the presence of possible transgenic contamination.
Case Study: Dicamba Incident
In 2016, the United States Environmental Protection Agency (EPA) approved the use of a formulation of an herbicide called dicamba for use with dicamba-tolerant transgenic soybean and cotton. In 2017, historically unprecedented crop damages due to dicamba drift/volatilization were reported, covering an estimated 3.6 million acres (Center for Food Safety, 2018). Probably no other recent case more vividly demonstrates the potential for unintended consequences of agriculture biotechnology associated with a transgenic crop.
The likely cause of this unprecedented crop damage is the capacity of dicamba to volatilize (vaporize) from soil and plant surfaces for several days after application. These vapor clouds can then drift over long distances and cause destruction or damage to other crops not resistant to dicamba. Other causes could have been the failure of commercial pesticide applicators and/or farmers to fully understand detailed application instructions provided on labels of dicamba-based products and failure to adequately pre-test the capacity of these new formulations of dicamba to volatize and drift long distances.
As of this writing, the EPA has imposed new requirements for dicamba labels and usage, changed the status of dicamba to a restricted-use pesticide requiring application by licensed applicators only, and imposed other recordkeeping requirement for the 2018 crop year. Four states—Arkansas, Missouri, North Dakota, and Minnesota—have established cut-off dates after which dicamba cannot be used. Two more states, Indiana and Tennessee, have enacted additional use restrictions.
Concentration of the GMO seed industry often makes it difficult for certified organic farmers to obtain non-GMO seed. The Organic Seed Alliance is a national nonprofit that provides detailed information for organic farmers about accessing certified organic seed and how to produce seed for their own use and breed new varieties adapted to local/regional ecologies.
Gene editing has also created a new set of challenges for the organic crop industry. First, using cisgenic crops is not accepted by the USDA National Organic Program and, therefore, is a violation of organic certification. This is true for organic certification programs worldwide. Thus, purchasing certified organic foods can provide one means of assurance of food free of genetic modification, including cisgenic crops. However, detection of cisgenic crops may be more complicated and as yet is not regulated world-wide. Labeling programs like Non-GMO Verified have recognized gene editing efforts and will likely continue to include cisgenic crops as GMO.
Industry Concentration of Seed Ownership
The broadening of intellectual property rights in 1980 to cover living organisms, including genes, has resulted in a flurry of mergers and acquisitions in the biotech seed and agrichemical industries. According to an early (2001) Wallace Center report, “Relatively few firms control the vast majority of commercial transgenic crop technologies.” These firms have strategically developed linkages among the biotechnology, seed, and agrichemical sectors to capture as much market value as possible.
As of 2011, three companies controlled more than half of the proprietary biotech and non-biotech seed market. In 2014, six major agrichemical companies controlled much of the agrichemical, transgenic, and non-transgenic seed crop industry (Howard, 2015). However, by 2016 there was even further concentration, leading to a possibility of four companies exerting major market dominance of the transgenic crop market, pending U.S. government approval of a merger between the Bayer and Monsanto corporations.
Figure 2 provides a graph showing the change in the concentration of the global seed market from 1985 to 2015. The top five (C1-C5) seed companies control close to 55% of the global seed market.
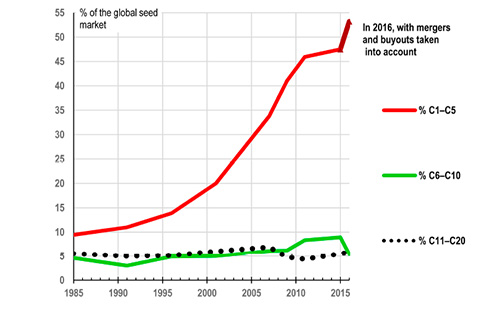
Figure 2. Concentration of the Global Seed Market. Source: Bonny, 2017
The next tier of companies, six through 10, (C6- C10) and the top 11 through 20 companies control about another 5% each. These tightly controlled linkages of seed and other product sectors raise serious issues of anti-trust law, market access, product innovation, and the flow of public benefits from transgenic crops (Wallace Center, 2001).
A major cause of this concentration in the transgenic crop industry has been continued legal protection under what is known as the intellectual property rights associated with genetic patenting, which amounts in practice to exclusive utility patents. Unlike plant-variety protection—which does not allow for the patenting of individual genes, but only of crop varieties—the form of intellectual property rights conferred to biotech firms holding patents for transgenic crop cultivars can prohibit farmers from saving seed and undertaking their own breeding programs, as well as prohibit plant breeders from using the new genetic material to create new generations of varieties adapted to specific regions or growing conditions (Guebert, 2001). Table 1 shows the legal history of transgenic-crop intellectual property rights history from 1930 through 2013. This legal history, along with industry concentration, has limited farmers’ counter rights to save seed.
This industry concentration and intellectual property rights history has many potential positive and negative consequences. Sylvie Bonny, in a recent research article, suggested the following positive and negative consequences (2017):
Potential negative consequences:
- Higher seed prices
- Decrease in diversity of the seed supply
- Loss of cultivated biodiversity
- Increased intellectual property rights
- Increased economic power of the largest companies over others, through licensing agreements
- Control over the seed and agrichemical industries and concentration of resources
- Promotion of corporate interests with public authorities and elected officials, which gives large companies a strong political influence
Potential positive consequences, through genetic seed/crop modification, include:
- Enhanced ability to adapt to and cope with climate change
- Better tolerance to drought or salinity
- Improved efficiency of nitrogen absorption
- Fortification of food in some human-health components
Despite these economic, political, and social-equity issues, several organizations have made attempts to improve historical government efforts to maintain and develop public breeding programs and crop cultivars. For instance, the National Sustainable Agriculture Coalition (NSAC), with some 50 representative members, has a long history of developing public policy on this issue.
Finally, the adoption of transgenic crop varieties has brought with it an increasing prevalence of contract production. Although contract production can lead to increased value and reduced risk for contract holders, farmers are justified in their concerns about loss of control when they sign a contract with a private company. Issues associated with contract production of transgenic crops, such as ownership, control, and social equity, must be considered within the broader context of sustainable agriculture.
Regulation of Genetically Modified Crops
Much of the controversy over transgenic crops, both internationally and domestically, is in part a result of how the United States regulates transgenic crops. The Federal government has determined that the commercial products of agricultural bio-technology are “substantially equivalent” to their non-GMO counterparts and that, therefore, no significantly new regulatory process or structure is needed for their review and approval.
Currently, three Federal agencies regulate the release of transgenic food crops in the United States: the U.S. Department of Agriculture’s Animal and Plant Health Inspection Service (USDA–APHIS), the U.S. Environmental Protection Agency (EPA), and the U.S. Food and Drug Administration (FDA). In the past few years, there has been increased effort to “modernize” and better coordinate the regulatory process between these three agencies. This led to the publication of the 2017 updated final version of the Coordinated Framework for the Regulation of Biotechnology (EPA, 2017). This document provides great detail on the transgenic crop regulatory process. The following provides a general overview.
USDA–APHIS
APHIS looks at how a transgenic plant behaves in comparison with its unmodified counterpart. Is a transgenic plant as safe to grow as an unmodified counterpart? More specifically, are agricultural plants and agriculturally important natural resources going to be damaged by use of transgenic cropping systems? Damage in this context has a very specific meaning that is limited to biological, chemical, and/or physical damage (EPA, 2017).
EPA
The EPA regulates the pesticides produced by transgenic crops, such as the Bt in Bt corn and cotton. It does not regulate the transgenic crops themselves. The goal is for the EPA to prevent unreasonable adverse effects of pesticides on the environment. This includes assessing economic, social, and environmental risks and benefits from pesticides produced by transgenic crops. It also specifically includes assessing the safety of a pesticide and its related compounds “for dietary or residential human health effects” (EPA, 2017, p. 9).
FDA
The FDA focuses on the human-health risks of transgenic crops. However, its rules do not require mandatory pre-market safety testing of transgenic foods. Instead, a voluntary pre-market consultation process has been established, which includes reviewing possible issues of potential allergenicity and toxicity of the transgenic plant used as human or animal food. If the voluntary pre-market consultation was not undertaken, the firm marketing a transgenic-based food product continues to have the responsibility to ensure that their products are safe and lawful (EPA, 2017). Initially, the U.S. regulatory process for transgenic food crops required product-by-product reviews. Now, however, to simplify and speed up the process, new products can be approved based on the experience gained in reviewing earlier products.
Central to the policy of substantial equivalence is the assumption that only the end product of transgenic technology is of concern—not the process of genetic modification. Canada has adopted a similar approach. Europe and other U.S. trading partners, however, have taken a more conservative approach. They focus on the process of genetic modification—the source of many environmental and human-health risks that are of greatest concern.
How these different approaches play out in reality can be summed up simply. The United States and Canada assume a genetically modified product is safe until it is proven to carry significant risk; the European Union, which follows the “precautionary principle,” assumes the same product may carry significant risk until it can be proven safe. The science used by the two approaches is not fundamentally different. The difference is in the level of risk the different societies and political systems are willing to accept (ESCOP/ECOP, 2000).
Gene editing and other “new genetic modification techniques” (NGMTs)
As mentioned earlier, gene-edited and/or other non-transgenic (cisgenic) crops are newly introduced into commercial markets. While there was an initial attempt to provide some regulation of cisgenic crops, as of this writing (2018), these crops will be treated by USDA-APHIS as equivalent to crops created through traditional breeding practices. Because most current cisgenic crops are not “pesticidal,” the regulatory authority for involvement of EPA has not until recently been important. The exception is a new pesticidal corn seed called DvSnf7 dsRNA that acts in a way similar to Bt corn, using a NGMT called RNAi (interference). The companies that have just recently released cisgenic crops have all gone through the voluntary premarket consultation process with FDA.
Labeling of Genetically Modified Food
After many years of civil-society policy efforts and the passage of several state laws, the National Bio-engineered Food Disclosure Standard was signed into Federal law in 2016. This law is intended to establish a national labeling standard for genetically modified food and seeds. This disclosure standard began implementation in January of 2019. The law is only for genetically modified foods, with meat, poultry, and egg products requiring disclosure/labeling in limited circumstances. Under this law, the manufacturers of bio-engineered food can choose from three methods of disclosure: text, a symbol, or an electronic/digital link. Small food manufacturers will be exempt from the law.
Concluding Remarks
Implications for Sustainable Agriculture
In contrast to the ecological approach of sustainable agriculture, current transgenic crops follow a single weed- and insect-pest-management model that does not significantly vary from the chemical pest approach. This approach has led to a similar outcome: the development of weed and pest resistance to the transgenic crop (Wallace Center, 2001).
Standard plant-breeding methods can potentially solve many of the same problems in agriculture that transgenic crop engineers are working on. For example, the Mandaamin Institute in Wisconsin has used classical in-field plant breeding and selection techniques to develop highly nitrogen-efficient and nitrogen-fixing field corn. Its advanced breeding lines give yields equivalent to modern conventional and GMO cultivars, with superior protein percentage and quality and a capacity to maintain yields in organic and reduced-input systems, during drought, and in lower-fertility soils (Mandaamin Institute, 2018). In addition, while the average cost to develop a new GMO cultivar and bring it to market is more than $100 million, an investment of just $27 million in USDA research dollars in several farmer-participatory classical breeding endeavors has led to the release of more than 40 new crop cultivars and hundreds of advanced breeding lines (Schonbeck et al., 2016).
Yet, advanced laboratory techniques such as genomic analysis (DNA sequencing) can enhance the efficacy of traditional plant breeding in attaining some plant-breeding objectives. For example, armed with the map of an organism’s genetic code, scientists can identify which genes are in a plant cultivar, breeding line, or accession, and can more easily select which cultivars or lines to cross-breed to obtain the desired suite of traits. “Before we knew where the genes were, we were still breeding in the dark,” according to Steven Briggs, head of genomics for Syngenta, a Swiss biotechnology giant, as quoted in The New York Times (Pollack, 2001).
Gene editing and other NFMT methods of crop (and animal) genetic modification, however, pose new issues that may have both positive and negative impacts on agricultural sustainability. Gene editing and NFMT methods promise to provide crops that will address agriculture-sustainability issues such as crop adaptation to climate change, drought, heat stress, nutrition, nitrogen uptake, and others not yet imagined. Recent research on a gene-edited wheat that focuses solely on yield improvement will likely continue controversy around these new gene-modification techniques as well, possibly leading to the first genetically modified wheat released commercially. Importantly, because the focus is on yield improvement, this will not be associated with the coupled use of herbicide or pesticides (Dixon et al., 2018; Wang et al., 2018).
Gauging a Technology’s Impact on Agricultural Sustainability
The sustainability of any agricultural technology can be gauged in part by answering a series of questions that emerge from the basic principles of sustainable agriculture. Farmers can ask themselves these questions in the context of their own operations to help determine whether adoption of this technology will move them away from, or toward, increased sustainability:
- Does the technology increase genetic diversity?
- Does it maintain a positive balance of pests and predators?
- Does it protect and enhance important soil biota for plant growth and health?
- Does it decrease the quantity or concentration of toxins released into the environment?
- Does it decrease soil erosion?
- Does it protect non-target organisms?
- Does it help protect natural habitats?
- Does it reduce pest populations and viability?
- Does it increase farmers’ yields and/or lower farmers costs?
- Does it increase farmers’ market control? Management flexibility? Time?
- Does it provide benefits to consumers? Will consumers accept it?
- Does it help citizens globally gain better access to food?
- Does it protect the public’s access to information and improve public trust in agriculture?
- Does it protect or enhance the food security and food sovereignty of the communities in which the technology will be implemented, and in which the resulting foods will be sold?
If the answer to any of the above questions is no, then a cautious approach to the adoption of the technology in question would seem in the interest of agricultural sustainability.
Technology and the Public Good
Evelyn Fox Keller, author of The Century of the Gene (2000), describes the scientific understanding of genetics that originated with the discovery of DNA in 1953, and on which the current generation of genetically modified crops is still based:
“For almost fifty years, we lulled ourselves into believing that, in discovering the molecular basis of genetic information, we had found the ‘secret of life’; we were confident that if we could only decode the message in DNA’s sequence of nucleotides, we would understand the ‘program’ that makes an organism what it is.”
Recent scientific discoveries no longer support this belief. Genetic modification of crops will not result in some single “yield” gene modification that will solve the complex issues of world poverty and hunger. Keller, a professor of History and Philosophy of Science at Massachusetts Institute of Technology, insists that the most recent scientific understanding of genetics has more to tell us about biological organization than about how to modify individual traits. While the new leading-edge biotechnology is striving to capture the benefits of genetic engineering without the costs and risks of potential genetic instability, the economic, political, and social costs and benefits of this technology are far from being fully understood.
Molecular biologists and breeders, and the new techniques of gene editing, are beginning to utilize the emerging knowledge of gene location and function to guide them with growing precision. This approach will likely supplement or overtake the transgenic crops of the present. Is this good news for sustainable agriculture, which is based on understanding how natural systems work in order to fit human enterprises into them?
According to Fred Kirschenmann, organic farmer and former director of Iowa State University’s Leopold Center for Sustainable Agriculture:
“The real benefit of genetics seems to be derived not from the manipulation of a few genes, but from our enhanced understanding of how nature works” (2001).
Regardless of the future direction of crop genic technology, one thing remains certain: many of the unresolved issues for farmers, ranchers, and the general public will not be settled, nor should they be settled, through the use of biological or natural sciences alone.
Further Resources
International Service for the Acquisition of Agri-biotech Applications (ISAAA) Database
The National Agricultural Law Center: Biotechnology
Genetically Modified Crops: Transgenics and Cisgenics
By Nancy Matheson and Katherine Adam, NCAT Agriculture Specialists
Published 2006
Updated by Jeff Schahczenski, NCAT Agricultural and Natural Resource Economist, June 2018
IP189
Slot 172
Version 052019
This publication is produced by the National Center for Appropriate Technology through the ATTRA Sustainable Agriculture program, under a cooperative agreement with USDA Rural Development. ATTRA.NCAT.ORG.