Geothermal Greenhouses: Exploring the Potential
By Victorian Smart, NCAT Energy Program Assistant; Danielle Miska, NCAT Energy Engineer; and Katie Simpson-Johnson, Energy Corps
Abstract
This publication provides basic information on geothermal energy technology and its potential in greenhouses. It explains how geothermal heating and cooling systems may expand the features of a growing structure while maintaining minimal energy usage. This publication discusses active and passive ground-source geothermal energy systems and provides design examples for passive system design suited to agricultural operations.
Introduction: Geothermal Energy for Agricultural Growing Structures
Agricultural growing structures, such as greenhouses and high tunnels, are used for plant propagation, season extension, and to enhance and control crop production. Greenhouses and high tunnels can have the same basic structure: metal frames and plastic polyethylene, polycarbonate, or glass walls and roof. Both provide varying degrees of temperature and climate control to extend the growing season. High tunnels, sometimes called hoop houses, differ from greenhouses in that they include non-automated climate controls (like vents or roll-up sides) and are typically used for season extension rather than year-round growing. Traditional greenhouses are primarily used for plant propagation, require heat usually provided through automated climate controls, and generally built with more durable materials. This also allows for more consistent year-round growing. A third variation, passive solar greenhouses, consists of structures typically smaller than high tunnels that do not use power-generated heat sources like greenhouses do.
Traditional greenhouses protect crops in temperature extremes by using temperature, humidity, and ventilation controls to maintain an ideal climate (Rimol Greenhouse Systems, 2019). Generating such a climate can result in significant energy costs. Heating and electric combined is typically the third-largest cost for operating a traditional greenhouse (Penn State Cooperative Extension Service, 2020). By contrast, high tunnels and solar greenhouses are passive in design and do not require extra energy for heating and cooling, making them a more cost-effective option for certain operations. However, growers can further benefit from these structures when they are supplemented with an equally cost-effective climate-control system. Perhaps surprisingly, an efficient source of supplemental energy can be found right below our feet.
Renewable geothermal energy is heat derived from the subsurface of the earth (EPA, 2016). Underground temperature becomes stable starting roughly two feet below the earth’s surface and continues to stabilize at deeper depths. Underground soil maintains a constant temperature year-round, averaging 55°F throughout the United States. Geothermal systems allow us to take advantage of these stable temperatures. In general, a system is comprised of two main components: underground tubing or piping to support the flow of a fluid (either air or a glycol solution) and the mechanism to push the air or fluid in and out of the piping. These primary components work to turn over the air in the structure to meet heating or cooling needs. Movement of air or fluid is achieved in various ways, such as using a heat pump or a simple inline fan.
This publication provides basic information on geothermal energy technology and its potential in greenhouses. It explains how geothermal heating and cooling systems could expand the features of a growing structure while maintaining minimal energy usage. This publication focuses on two types of ground-source geothermal energy systems, one of which is more sophisticated in design and requires a heat pump. The other is a more “passive” system that is similar in design but does not require a heat pump. Lastly, it provides design examples for a passive greenhouse system design that best suits agricultural operations.
How Geothermal Systems Work
The flow of heat energy within a geothermal system can be utilized to heat or cool a space, depending on seasonal needs. Air is moved from inside a building and pushed through a network of underground piping. Convection through this piping heats or cools the air, which is then exhausted back into the structure at a more desirable temperature. When the underground temperature is warmer than the ambient temperature above ground, the heat pump or fan pushes the warmer air up to the growing structure that needs to be heated. When the temperature in the growing structure is too warm, the pump or fan removes the warm air from the building and pushes it into the pipe below ground, returning cooler air to the building (Figure 1).
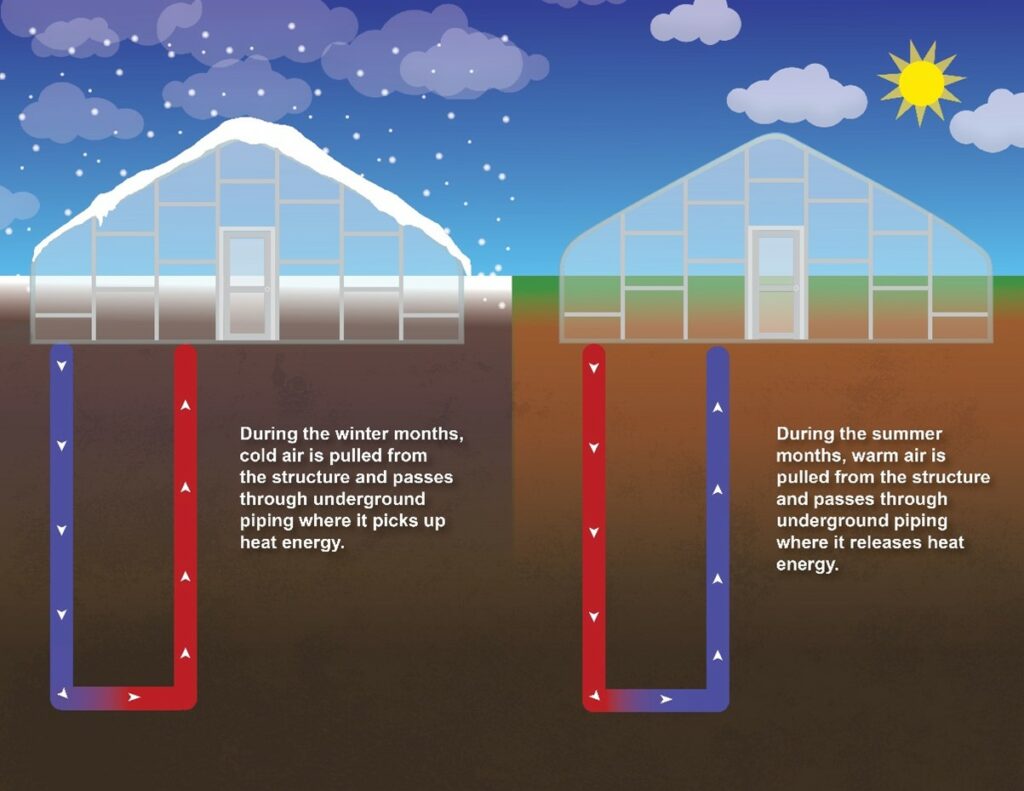
Figure 1. Flow of Heat Energy in Geothermal System. Graphic: Marisa Larson, NCAT
Active Systems
An active system utilizes a water-to-air heat pump, a liquid (either water or a glycol solution) as the medium for heat exchange, and evaporator and condenser coils. It uses the basic refrigeration cycle of evaporation, compression, condensation, and expansion to heat or cool the air before it is delivered to the space. The piping can be organized in the ground horizontally (trenches) or vertically (bore holes). Horizontal trenches are most applicable for small-scale operations because a vertical system requires drilling several deep holes (about 200 to 500 feet) to install the piping. This can become expensive because of the deep drilling that is required, although it does have greater ability to offset energy needed for heating or cooling in more sophisticated buildings and large operations (EPA, 2016). In this system, the liquid runs through piping in the ground, either absorbing or diffusing heat, depending on the temperature needs of the building above.
If the property has a private water source such as a pond, lake, or well, other options are available that do not require excavation. In these systems, water is pumped into the piping from the pond, for example, and this functions as the medium for heat exchange. These are active systems because they use a heat pump to pump the water from the water source into an exchanger, which heats or cools the air and then sends it into the building.
Standard geothermal heating and cooling systems are rated on their coefficient of performance, as a ratio of a product’s heat output to the electrical energy input required (Kamoshida et al., 1990). Standard systems have efficiencies of 300 to 600%. Compare this to the efficiency of a standard fossil-fuel furnace, which varies from 75 to 90% (ASHRAE, 2018). “Geothermal systems are [at least] twice as efficient as the top-rated air conditioners and almost 50% more efficient than the best gas furnaces, all year round” (Alexander, 2018).
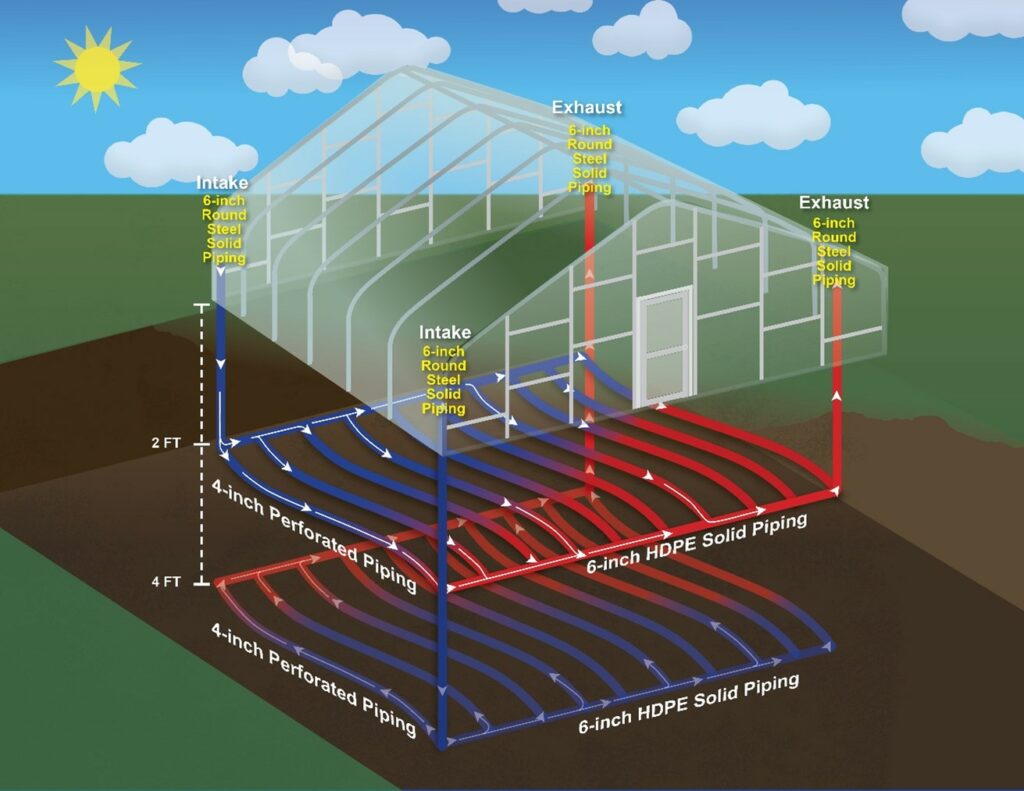
Figure 2. Example design of groundwork in a passive geothermal system. Two layers of piping are depicted here, showing the flow of heat energy. As cool air is being pulled in, warm air is being pushed out. This is organized so the flow in one layer moves inversely to the other layer. Graphic: Marisa Larson, NCAT
Passive Systems
A passive geothermal system is often called an earth battery, climate battery, or low-grade geothermal system. This system is comprised of piping, risers, manifolds, fans, and the insulated mass of soil. It uses the same fundamentals of thermodynamics as a ground-source system with a heat pump but has less intensive installation, which significantly reduces cost and requires only the energy needed to keep a fan or two running. Fans blow hot air from the growing structure into perforated piping that runs underground. When warm air is pushed into the piping below ground, the heat in the air traveling through the tubing is allowed to diffuse throughout the soil. This “charges” the soil with warm air that can later diffuse back into system (Osentowski and Thompson, 2021).
These passive systems rely on perforated tubing coming into contact with as much soil as possible for the maximum energy exchange to occur. Many of these systems are modified by growers who layer piping at two and four feet, or four and six feet, or six and eight feet. This piping must be organized horizontally for the system to function properly and for the growing structure to maximize its efficiency (Figure 2). Rigid foam insulation running around the perimeter of the underground portion of the system is necessary to maximize a passive system’s output. This system also functions with no heat exchanger, as the cooled or heated air simply blows out of the other end of the piping, back into the growing structure.
Geothermal Applications
Passive Solar Greenhouse
Although a passive solar greenhouse typically requires little to no energy input for climate control, using a passive geothermal system can further improve the season extension utility of the greenhouse and allow for diversity in crop production. An active system would provide the same benefits, but it would reduce the passive solar greenhouse’s energy efficiency. Passive solar greenhouses tend to be small, therefore holding a small volume of air. The passive system functions most efficiently with smaller volumes of air and therefore would not need the amount of energy produced by an active system. A length-width ratio of 2:1 for any structure is ideal for energy efficiency (Bradford Research Center, no date).
Traditional Greenhouse
Traditional greenhouses are often larger than passive solar greenhouses and would most likely not be adequately heated or cooled by a passive system. An active system would be capable of providing adequate heating and cooling for greenhouses of various sizes with an appropriately sized heat pump and enough linear feet of underground piping. As well, a greenhouse of substantial size may provide the necessary payback from crops to make the expensive installation of an active system feasible. Some large-scale commercial growers have many acres of greenhouses. These settings would be most appropriate for installing active systems, because such a grower would likely see a faster payback on the investment.
High Tunnel
Heating or cooling a low-cost structure such as a high tunnel with an active system would not be cost-effective because of the tunnel’s less-sturdy design with intended use of season extension only. Both the high tunnel structure and the passive system are low-cost options; however, a high tunnel is typically constructed with minimal insulation. Because the design is minimalistic, with one layer of plastic covering, climate control with a passive geothermal system would not be sustained for very long. In seasonal extremes, the “battery” that is the passive geothermal system will eventually reach its full capacity and will no longer heat or cool the space. Consequently, the passive, climate-battery system is the most feasible ground-source geothermal system for a small-scale grower, but it is primarily suited to a passive solar greenhouse or a double-walled, insulated high tunnel.
Before You Build
When designing a geothermal system, first consider your hardiness zone (USDA, 2012), the temperature variance your area experiences throughout the year, and what your temperature needs are for your crops. You should also assess the value of the additional produce the season extension would afford. Consider the marketability throughout the growing season and whether it is worth the investment of finances and labor for heating and cooling a structure, especially a low-cost structure, such as a high tunnel. Other questions to ask yourself include these:
• What are my heating and cooling needs?
• Which technologies can provide the necessary heating and cooling energy?
• How do renewable heating and cooling options compare with conventional technologies?
• What renewable resources are cost-effective in this part of the country?
• What renewable heating and cooling products should I choose?
• Who will install and maintain my system?
• How will I finance the system?
• Does the value of additional produce offset this cost?
• How much value is there in reducing the risk from freezing or overheating?
• What if fossil fuels are cheaper?
Energy Efficiency
The grower must first ensure that a structurally sound and well-insulated building is capable of keeping air inside, before installing a geothermal heating and cooling system. Otherwise, the effort and expense is wasted. In general, growing structures are more effective when constructed on well-drained, level land. Do not construct where the water table is known to be high or where you have an impermeable soil layer. Growing structures should be oriented to maximize sunlight and with end walls facing the winter winds. If these two requirements contradict, experts advise that the structure should capture as much winter sunlight as possible. It is also important to build the structures twice as far from any potential shading obstacle as the height of that obstacle (USDA, 2014).
It is necessary to seal a high tunnel to all ambient air, so it may be heated adequately by the geothermal system. Construct your high tunnel with a double-layered wall of greenhouse plastic and inflate the space between with a blower fan to enhance insulation. As well, use twin-wall hard covering for end walls. This kind of rigid covering performs better than simple greenhouse plastic in strong winds and requires less maintenance over time. Seal or insulate doors, vents, or shutters when the high tunnel is not in use. These openings may appear tightly sealed when shut, but they are typically where these structures experience a huge loss of energy. One way to overcome this is to seal these openings with scraps of greenhouse plastic. Seal the base perimeter of the high tunnel and use frost blankets to cover crops inside the high tunnel. It is also important to install ventilation in these structures, to let in cooler air during the warm months. Many high tunnels utilize roll-up sides for ventilation during warmer months, so any type of sealing done during cold months needs to be temporary. Maximizing the efficiency of the growing structure prior to geothermal installation will only serve to maximize profits (Alexander, 2019).
Follow similar rules for constructing a greenhouse. Do not construct where you know the water table is high or where the land experiences ponding. Construct to maximize sunlight and to face end walls into the winter winds. Choose the appropriate style, doors and hardware, covering material, and ventilation system to suit your growing needs (Rimol Greenhouse Systems, 2020). Design experts advise that environmental controls be set up alongside the geothermal heating and cooling system to maximize the structure’s energy efficiency.
A modification of the traditional greenhouse is the passive solar greenhouse. This type of greenhouse does not use an artificial heat source but, rather, stores solar heat within a medium to be released at colder periods, like at night or during cold months. Storage mediums are typically black barrels filled with water, situated along the north wall. Alternatively, the entire north wall can be made of concrete to create an exceptional thermal mass. The north wall is much taller than the south wall in this design, to maximize southern sun exposure to charge the storage mediums. Although the use of barrels is popular, it is worth investigating for your operation because their heat storage capability is questionable, and they take up much space that could otherwise be used for crop production. This style of greenhouse has a large, angled, south-facing wall, covered entirely in greenhouse plastic, glass, or another transparent material. Additionally, east- and west-facing inner walls can be lined with a reflective material. Pitch of the roof and overall orientation of a passive solar greenhouse are primary components of effective design and should be considered, as well as carefully calculated, to maximize efficiency for the grower’s latitude. All considerations for traditional greenhouses, from location to hardware and additional systems, are necessary for this type of greenhouse, as well.
Unless the greenhouse or high tunnel is exceptionally well-insulated, significant heat loss will inevitably occur, especially in colder climates. This loss can exceed the capacity of the passive system itself. To prevent depleting the underground soil of its stored heat energy and causing the average, stable temperature to drop below 55ºF, experts recommend shutting off the climate battery and using an alternative heating method during extended periods of extreme cold (Osentowski and Thompson, 2021).
Energy Needs
Conducting an energy assessment of an existing growing structure can provide you with a better understanding of current energy usage and reveal inefficiencies that may have gone unnoticed (Penn State Cooperative Extension Service, 2020). Improvement of on-farm energy efficiency can only occur from a foundational understanding of the current operation. You may hire a professional energy auditor or use an energy calculator as a “do it yourself” alternative. In an assessment, consider your building structure, its structural soundness, and any energy conservation measures (ECMs) that you may already have in place. The shape of the frame, the length of the structure, height of side walls, layers of plastic poly-film covering, air pockets between a double-layered wall, ventilating roll-up walls, energy curtains (inside of the structure), shade curtains (on top of the structure), and row coverings are all ECMs that can contribute to reaching the desired temperature for growth. If you utilize some or many of these measures, then consider a smaller geothermal system to supplement them. If these measures are not implemented yet in the operation, consider how they can be paired with this type of heating and cooling system to increase efficiency and further extend the growing season.
As part of a conference presentation, Milton Geiger from the University of Wyoming provided this calculation for heating requirements in a building:
Square feet of structure surface X temperature difference (inside temperature minus outside temperature) X 1.2 = Heat needed in BTU/hour
Design and Installation
Despite the relatively simple design of geothermal systems, having the right plan in place prior to installation is essential, as small mistakes could undermine the effectiveness of the system. Important preliminary steps are to ensure that tubing or piping size is appropriate for the structure and that the organization of tubing or piping is secure before the backfilling stage occurs. Hiring an excavation service is expensive and excavating the pit yourself is time consuming, so re-excavation to modify the underground system should be avoided. According to Ceres Greenhouse Solutions, fan size, pipe diameter, pipe length, proper sealing in the infrastructure, drainage, pipe blockage, fan operation in humid environments, and how long the system runs are all things to consider and adapt to the grower’s operation (2015). Pipe diameter, pipe length, and air flow interact with each other and should be balanced in order to determine how much energy will be transferred between air and soil, how much air flow the grower will see, and ultimately the total heating/cooling capacity of the system (Ceres Greenhouse Solutions, 2015). Additionally, piping used for the passive system should be perforated. Condensate naturally occurs from the evaporative cooling process, so perforated piping is important to prevent water from collecting and plugging the pipe.
Appropriate sizing of hardware and equipment is crucial for gaining optimal efficiency from a geothermal system. Particularly with a low-grade/earth-battery design, the pipe size and fan power should match the volume of the growing structure and therefore produce a certain number of air turnovers per hour. Five air turnovers per hour is the ideal rate for reaching optimal efficiency for any greenhouse or high tunnel (Osentowski and Thompson, 2021). Fans used in this system should generate enough cubic feet per minute (CFM) to reach five turnovers. Some calculating is needed to determine this:
Volume (cubic feet) = CFM needed for system
Exchange rate (minutes)
Note that a fan with a higher flow rate is going to be significantly higher in cost. Consider fan options that are rated for outdoor use because they are more likely to withstand the operating environment of an agricultural structure.
For maximum benefit from a climate-battery system, it should primarily run only when excess heat is in the structure or any time the structure temperature is lower than subsoil temperature. Use a two-stage thermostat, or an integrated control system if other automated systems are being controlled, in order to avoid depleting the climate battery of its stored heat. When the structure’s temperature becomes equal to the subsoil “battery” temperature, heat transfer is no longer occurring; therefore, the fans do not need to be running.
The installer should note that because of the design of the passive earth-battery system and its interaction with surrounding soil, it is crucial that the area in which the underground tubing is laid is insulated around its perimeter. Two-inch-thick rigid foam insulation serves to contain the heat stored underground and prevents heat energy from being leached into the cooler surrounding soil. Additionally, soil type plays a role in installation and maintenance of this system. Sandy, loam soil is ideal for this, not to mention its effectiveness for plant growth. Too much clay in the soil can harden around the tubing from the wetting and drying cycles, eventually plugging the system. If your soil is high in clay, consider replacing the soil during excavation or amending the soil to lower clay content (Osentowski and Thompson, 2021).
Hot air flowing through the pipes in a passive system will heat up the soil for about a foot surrounding the piping on all sides. Consequently, allow two feet of space between each row and each level of piping to ensure the soil is “charging” at its maximum capacity. If you are able to install a geothermal system and its groundwork prior to installing the actual high tunnel or greenhouse, the piping or tubing system would sit directly underneath the structure. If a structure is already in place, the underground piping for a retrofitted geothermal system should be buried next to the north or south-facing wall. This system would still require the same amount of linear feet of piping to be effective, and this pipe also needs to be spaced two feet away from the next pipe on all sides. Below, we will further explain calculating energy needs and the expense of installing a low-cost version of a geothermal system.
Costs and Payback
Remember to include operating costs in project cost calculations. A small amount of energy is needed for the heat pump in an active system, to drive the heating and cooling processes, but as much as five times this much energy is being brought up from the ground, resulting in a net gain of energy for the system. Fans in a passive system need even less energy to run.
Cost can vary widely, depending on system mechanics and design. Parts and installation can range from $1,200 to $25,000, depending on the system. There can be significant variation in upfront costs because, as this technology becomes more understood and utilized in agriculture, growers are developing and implementing passive systems that require less sophisticated equipment and fewer parts. Additionally, growers may already have access to equipment, like an excavator or tractor, whose rental or hire typically accounts for the majority of system installation cost. An example of costing a passive geothermal system is described in the first case study, below.
On the other hand, in an active system, the heat pump itself can represent as much as 60% of the system cost (Stimson, 2016). The size of the heat pump, as well as the design of the underground piping, will vary from one application to the next. Because of the sophistication of the system and extensive design requirements, an active system should be designed by an engineer and installed by a professional. A heat pump may further accommodate your seasonal and operational needs by providing you with a more direct means of climate control, provided it is financially appropriate. Contact your local HVAC equipment provider or a geothermal systems vendor for sizing and pricing.
Case Studies
Dave McCarson, Alpine Organic Farms, Montana
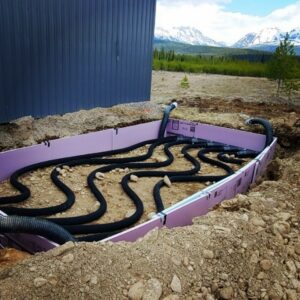
The two-foot layer of perforated piping and insulation in a passive system. Photo: Dave McCarson
Dave McCarson specializes in design and construction of passive solar greenhouses and has expertise in horizontal open-loop, ground-air geothermal systems. He explains that this low-cost, climate-battery type of system is particularly effective in agriculture and farming. Systems he has designed and installed are typically small, 10 feet by 20 feet, and are comprised of piping layered at two feet and at four feet deep in the earth. For McCarson’s own system, installation was cheap because he had excavation equipment available, and he has general knowledge of using the hardware. He has seen success with installations and overall efficacy of this type of system in Plant Hardiness zones 3 and 4.
McCarson suggests that unless a high tunnel has double-layered, insulated walls, any geothermal system would be cost-inefficient for this application. This is because a typical uninsulated high tunnel will quickly release heat energy. Conversely, geothermal systems can generate a significant amount of energy, and an active system will produce too much energy for a single-layered high tunnel and its crops. McCarson points out that the nature of a high tunnel is to become too hot in the summer but not stay warm enough for the winter. Without double-layered insulated walls, the same problem would continue, even with a geothermal system.
The dimensions of McCarson’s structure and the hardware used can provide a cost estimate. Ten feet by 20 feet by 8 feet (typical height at the ridgeline for a high tunnel this size) constitutes 200 square feet of floor space and 1,600 cubic feet of volume. Using the calculation above, 133 CFM from inline fan(s) is needed for this size structure to achieve five air turnovers per hour. To get cubic feet per minute, the structure’s volume in cubic feet is divided by 12 minutes, since we desire five turnovers per hour (12 x 5 = 60).
(1,600 cu ft) = 133 CFM needed for system
(12 mins)
The groundwork involves two layers of piping, one at four feet deep and the other at two feet deep, running the length of the structure. To create a bidirectional air flow between the two layers, two intake pipes (one for each layer) are situated at opposite ends of the structure and pull air from near the ridgeline. Four-inch perforated pipe is used for the lengthwise tubing. Six-inch perforated pipe is used for the manifolds at opposite ends of the system for each layer.
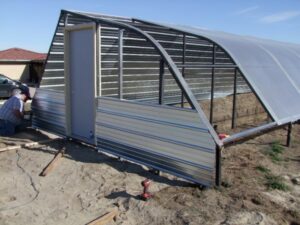
Construction of Finch’s passive solar greenhouse. Photo: Russ Finch
Russ Finch, Greenhouse in the Snow, Nebraska
Russ Finch specializes in passive solar greenhouse design complemented with open-loop, ground-source passive geothermal systems. The original unit attached to Finch’s home runs 78 feet long and 17 feet wide. However, this is smaller than the typical size of the units Finch commercially produces, which range up to 138 feet long, although efficiency is maximized at 102 feet. At his home greenhouse in eastern Nebraska, Finch grows large crops of citrus, figs, feijoa, grapes, and dozens of flower varieties. A typical lemon tree will produce 125 pounds per year, fetching $4.30 a pound at farmers market prices. It is notable that Finch is able to grow these crops in this part of Nebraska, an area that is rated at Plant Hardiness zone 4 or 5. In the winter, the temperature can drop to -27°F, and in the summer, it can climb to 105°F.
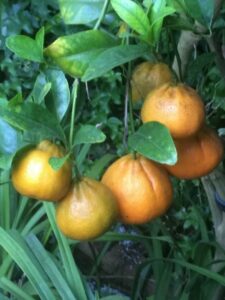
Citrus grown on Russ Finch’s operation. Photo: Russ Finch
Finch’s system utilizes the stability of soil temperature at eight feet below the earth. Along with this system, Finch also utilizes a unique passive solar greenhouse design that minimizes the volume of air that needs to be heated or cooled. Finch suggests that these systems are not feasible for hoop houses (high tunnels) or even for traditionally sized greenhouses. Both contain a large volume of air and conditioning this much air could potentially raise costs to the point where they would exceed the benefits of the system.
The greatest accomplishment of the systems Finch designs is their simplicity. The systems can easily be installed by anyone with access to a backhoe and the ability to do the labor. In an 8-foot-deep trench, extending from one end of the greenhouse to another, a series of perforated pipes are laid. Finch states that for every six feet of greenhouse length, an additional tube is required. For instance, a 96-foot greenhouse would require 16 tubes. Finch arrived at the numbers purely through trial and error and notes that it is difficult to set up hard calculations for this. This system regularly delivers a temperature difference of 35 to 40°F between the air being put into the ground and the air coming out. To develop estimates for your greenhouse and geothermal system, you can use an online climate battery calculator such as the one provided by Eco Systems Design.
Conclusion
The use of growing structures for season extension and the application of geothermal heat technology impact the sustainability of a growing operation. Unheated high tunnels can extend the growing season at minimal cost to the grower. With the help of structures that extend the growing season, the grower has more capacity for production and can grow their market and promote local food security. Adding a geothermal system can provide enhanced protection to crops and extend the growing season even further. Geothermal heating and cooling systems require very little dependence on conventional energy sources such as oil and gas, resulting in reduction of greenhouse gas emissions and air pollutants.
Geothermal energy is a renewable energy source, and with simple but durable design, a system can last a long time. A geothermal system is easy to maintain and requires little ongoing maintenance when built properly. There are many considerations regarding the economics of a geothermal system, but in some cases the investment can be worth it. A passive design variation in the form of a climate battery or other type of low-grade geothermal system is most feasible in agriculture when paired with an insulated high tunnel or passive solar greenhouse. Tapping into this renewable energy tool that is cost-effective and long-lasting can be invaluable for extending the growing season, increasing capacity for providing fresh produce, and contributing to the sustainability of food and energy systems.
Geothermal Greenhouses: Exploring the Potential
By Victorian Tilley, NCAT Energy Program Assistant; Danielle Miska, NCAT Energy Engineer; and Katie Simpson-Johnson, Energy Corps
Published November 2021
©NCAT
IP617
This publication is produced by the National Center for Appropriate Technology through the ATTRA Sustainable Agriculture program, under a cooperative agreement with USDA Rural Development. ATTRA.NCAT.ORG