Nematodes: Alternative Controls
By Martin Guerena, NCAT Agriculture Specialist
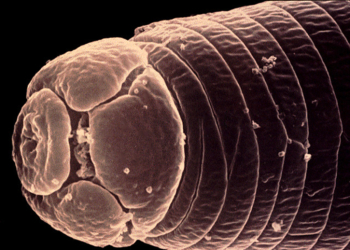
Root-knot nematode—Meloidogyne brevicauda Loos
Photo: Jonathan D. Eisenback, Virginia Polytechnic Institute and State University
Abstract
This publication provides general information on the tiny worm-like organisms called nematodes. A more detailed description of the genera of nematodes that attack plants is provided as well as various methods to diagnose, discourage and manage plant parasitic nematodes in a least toxic, sustainable manner.
Contents
Introduction
Symptoms and Sampling
Preventing Further Spread of Nematodes
Managing Soil Biology
Crop Rotations and Cover Crops
Botanical Nematicides
Biocontrols
Plant Resistance
Red Plastic Mulch
Solarization
Flooding
Summary
References
Further Resources
Web Resources
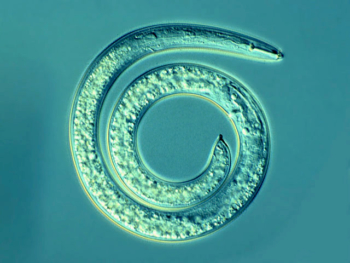
Spiral nematode, Helicotylenchus sp. Photo: Ulrich Zunke
Introduction
Nematodes are tiny, worm-like, multicellular animals adapted to living in water. The number of nematode species is estimated at half a million, many of which are “free-living” types found in the oceans, in freshwater habitats, and in soils. Plant-parasitic species form a smaller group. Nematodes are common in soils all over the world (Dropkin, 1980; Yepsen, 1984) As a commentator in the early twentieth century wrote:
“If all the matter in the universe except the nematodes were swept away, our world would still be dimly recognizable, and if, as disembodied spirits, we could investigate it, we should find its mountains, hills, vales, rivers, lakes and oceans represented by a thin film of nematodes.” (Sasser, 1990) |
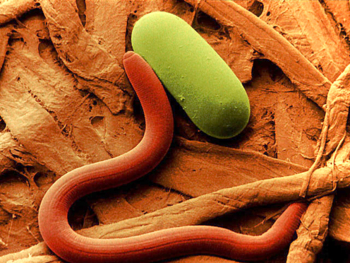
Pratylenchus sp. larva and egg. Photo: William Wergin
An important part of the soil fauna, nematodes live in the maze of interconnected channels—called pores—that are formed by soil processes. They move in the films of water that cling to soil particles. Many genera and species have particular soil and climatic requirements. For example, certain species do best in sandy soils, while others favor clay soils. Nematode populations are generally denser and more prevalent in the world’s warmer regions, where longer growing seasons extend feeding periods and increase reproductive rates. (Dropkin, 1980) In the southern United States, as many as ten generations are produced in one season. (Yepsen, 1984)
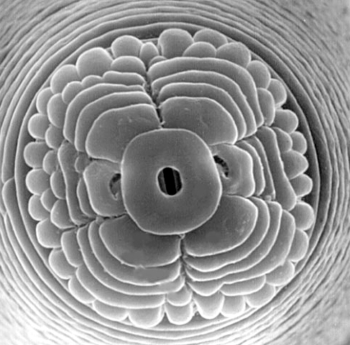
Face view of lance nematode, Hoplolaimus sp. Photo: Jonathan Eisenback
Light, sandy soils generally harbor larger populations of plant-parasitic nematodes than clay soils. This is attributable to the more efficient aeration of sandy soil, the presence of fewer organisms that compete with and prey on nematodes, and the ease with which nematodes can move through the root zone. Also, plants growing in readily drained soils are more likely to suffer from intermittent drought, and are thus more vulnerable to damage by parasitic nematodes. Desert valleys and tropical sandy soils are particularly challenged by nematode overpopulation. (Dropkin, 1980)
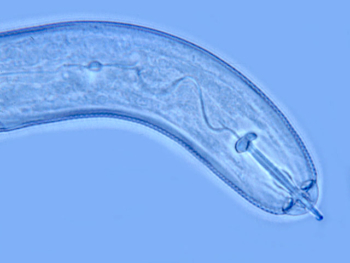
Sugarbeet cyst nematode juvenile. Photo: Michael McClure
Plant-parasitic nematodes—the majority of which are root feeders, completing their lifecycles in the root zone—are found in association with most plants. Some are endoparasitic, living and feeding within the tissue of roots, tubers, buds, seeds, etc. (Sasser, 1990) Others are ectoparasitic, feeding externally through plant walls. A single endoparasitic nematode can kill a plant or reduce its productivity, while several hundred ectoparasitic nematodes might feed on a plant without seriously affecting production. (Ingham, 1996) A few species are highly host-specific, such as Heterodera glycines on soybeans and Globodera rostochiensis on potatoes. (Sasser, 1990) But in general, nematodes have a wide host range.
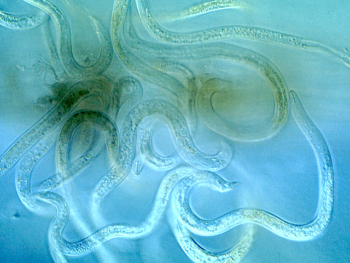
Lesion nematodes penetrating a root. Photo: Ulrich Zunke
Endoparasitic root feeders include such economically important pests as the root-knot nematodes (Meloidogyne species), the cyst nematodes (Heterodera species), and the root-lesion nematodes (Pratylenchus species). (Sasser, 1990) Important ectoparasitic root feeders include: root (Paratrichodorus and Trichodorus), dagger (Xiphinema), needle (Longidorus, Paralongidorus), ring (Criconemella, Macroposthhonia), stunt (Tylenchorhynchus and Merlinius), pin (Paratylenchus), and spiral (Helicotylenchus, Rotylenchus, and Scutellonema) nematodes. Direct feeding by nematodes can drastically decrease a plant’s uptake of nutrients and water.
Nematodes have the greatest impact on crop productivity when they attack the roots of seedlings immediately after seed germination. (Ploeg, 2001) Nematode feeding also creates open wounds that provide entry to a wide variety of plant-pathogenic fungi and bacteria. These microbial infections are often more economically damaging than the direct effects of nematode feeding.
Major Plant-parasitic Nematode Genera in the U.S. & Associated Damage to Plants
|
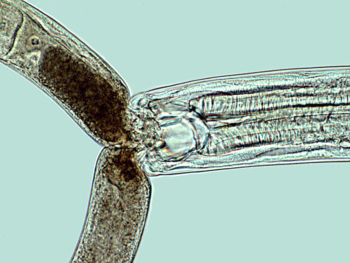
Mononchoid nematode feeding on another nematode. Photo: Jonathan Eisenback
Nematode control is essentially prevention, because once a plant is parasitized it is impossible to kill the nematode without also destroying the host. The most sustainable approach to nematode control will integrate several tools and strategies, including cover crops, crop rotation, soil solarization, least-toxic pesticides, and plant varieties resistant to nematode damage. These methods work best in the context of a healthy soil environment with sufficient organic matter to support diverse populations of microorganisms. A balanced soil ecosystem will support a wide variety of biological control organisms that will help keep nematode pest populations in check.
Symptoms and Sampling
Usually, sampling is done because the grower observes a section of field with unhealthy plants, or notices an unexplained yield reduction. Because nematodes damage roots, any condition that stresses the plant—such as drought (or even hot spells), flooding, nutrient deficiencies, or soil compaction—will tend to amplify the damage symptoms noted above. Failure to respond normally to fertilizers and slower-than-normal recovery from wilting are signs of nematode infestation. In the undisturbed soil of groves, turf, and pastures, visible symptoms of nematode injury normally appear as round, oval, or irregular areas that gradually increase in size year by year. In cultivated land, nematode-injured spots are often elongated in the direction of cultivation, because nematodes are moved by machinery. (Dunn, 1995)
It is important to note that species of nematode are present in all soils; their mere presence does not necessarily mean that they are damaging plants. Harmless or even beneficial species are found in proximity to plants, right along with the parasitic species. Beneficial nematodes feed on such pests as Japanese beetle grubs and plant-parasitic nematodes, and release nutrients into the soil by eating bacteria and fungi. (Ingham, 1996; Horst, 1990) An experienced nematologist can identify species, and determine which, if any, are responsible for the observed damage.
Nematode sampling techniques vary depending on the crop, the root depth, the type of nematode causing damage, and the time of the season. The procedure presented here is a generic sampling technique for annual crops. Soil samples taken in the late summer are best when testing for the presence of nematodes. Root-zone soil samples are best taken immediately after harvest, or just prior to harvest if the crop showed signs of damage. First, fields should be divided into 20-acre blocks that have similar damage, soil texture, or cropping history. From each block take several sub-samples, mixing them well to create a single one-quart sample for each block. Soil samples should be kept cool, but not frozen.
Samples for established perennial crops are best taken from the feeder root zone, which is usually located around the canopy drip line. (Dropkin, 1980) Your county or state Cooperative Extension Service can provide names of commercial labs that have nematode-identification services.
Preventing Further Spread of Nematodes
Preventing nematodes from entering uninfested areas is important; under their own steam they can spread across a field at a rate of three feet per year. The following measures will help prevent human-assisted spread of nematodes to uninfested fields:
- Use certified planting material
- Use soilless growing media in greenhouses
- Clean soil from equipment before moving between fields (washing equipment—including tires—with water is most effective)
- Keep excess irrigation water in a holding pond so that any nematodes present can settle out, pump water from near the surface of the pond; plan irrigation to minimize excess water
- Prevent or reduce animal movement from infested to uninfested fields
- Compost manure to kill any nematodes that might be present, before applying it to fields (Kodira and Westerdahl, 1995)
- Eliminate important weed hosts such as crabgrass, ragweed, and cocklebur (Yepsen, 1984)
Managing Soil Biology
The basis of sustainable nematode control is the maintenance of a healthy soil food-web. This begins with routine application of organic matter. There is substantial evidence that the addition of organic matter in the form of compost or manure will decrease nematode pest populations and associated damage to crops. (Walker, 2004; Oka and Yermiyahu, 2002; Akhtar and Alam, 1993; Stirling, 1991) This could be a result of improved soil structure and fertility, alteration of the level of plant resistance, release of nemato-toxins, or increased populations of fungal and bacterial parasites and other nematode-antagonistic agents. (Akhtar and Malik, 2000) Reduced nematode damage from increased organic matter in soil is likely a combination of these interaction. Higher organic matter content increases soil’s water-holding capacity, and supports thriving communities of the decomposers and predators that make up the soil’s “digestive system.”
Nematodes are important participants in this underground energy-transfer system. They consume living plant material, fungi, bacteria, mites, insects, and each other, and are themselves consumed in turn. Some fungi, for example, capture nematodes with traps, sticky knobs, and other specialized structures. (Dropkin, 1980) Nematodes and protozoa regulate mineralization processes.
Evidence suggests that between 30 and 50 percent of the nitrogen present in crop plants was made available by the activity of bacteria-consuming nematodes. (Ingham, 1996) Research in Denmark has indicated that nematodes convert about as much energy as earthworms in certain forest soils. (Dropkin, 1980) Don’t forget, the vast majority of nematodes found in the soil are not plant parasites.
The food web’s stability is challenged by the yearly turning of the soil, which reduces the numbers of organisms that displace or prey on plant-parasitic nematodes, while bringing more nematodes to the surface from deeper soil. If the same host crop is planted year after year, plant-parasitic nematodes may increase to damaging levels. Root-feeding nematodes are very opportunistic, and are among the first organisms to invade after a disturbance. (Dropkin, 1980; Ingham, 1996)
Keeping these facts in mind, it is important to actively manage soil biology using minimum-tillage practices, compost, animal manures, green manures, cover crops, and crop rotations. These practices help promote the growth of beneficial organisms while suppressing plant parasites. Certain organisms that are associated with well-managed crop soils—e.g., Rhizobacteria and mycorrhizae—may induce systemic host resistance to nematodes and to some foliar diseases. (Barker and Koenning, 1998) For further information see the ATTRA Topic: Soil.
Soil Amendments for Nematode Control
Some sources of organic matter known to be nematode-suppressive include oilcakes, sawdust, sugarcane bagasse, bone meal, horn meal, compost, and certain green manures. |
Most nematode species can be significantly reduced by tilling in chitinous materials such as crushed shells of crustaceans (shrimp, crab, etc.). This is effective because several species of fungi that “feed” on chitin also attack chitin-containing nematode eggs and nematodes. Increasing the amount of chitin in the soil will also increase the population of these fungi. A shrimp-shell-based fertilizer called Eco Poly 21™ Micro shrimp fertilizer is available from Peaceful Valley Farm Supply. At 2002 catalog prices, it would cost between $87 and $216 to treat an acre with this product (the suggested application rate is 20 to 50 lbs. per acre). Clandosan™, a nematicide made of crab shells and agricultural-grade urea, can be used as a pre-plant treatment (it should not be used on plants because the amount of urea in it can “burn” or kill them). (Fiola and Lalancettle, 2000)
Crop Rotations and Cover Crops
Crop rotation to a non-host crop is often adequate by itself to prevent nematode populations from reaching economically damaging levels. However, it is necessary to positively identify the species of nematode in order to know what plants are its host(s) and non-hosts. A general rule of thumb is to rotate to crops that are not related to each other. For example, pumpkin and cucumbers are closely related and rotating between them would probably not be effective to keep nematode populations down. A pumpkin/bell pepper rotation might be more effective. Even better is a rotation from a broadleaf to a grass. Asparagus, corn, onions, garlic, small grains, Cahaba white vetch, and Nova vetch are good rotation crops for reducing root-knot nematode populations. Crotalaria, velvet bean, and grasses like rye are usually resistant to root-knot nematodes. (Wang, et al., 2004; Yepsen, 1984; Peet, 1996) Rotations like these will not only help prevent nematode populations from reaching economic levels, they will also help control plant diseases and insect pests.
Allelochemicals are plant-produced compounds (other than food compounds) that affect the behavior of other organisms in the plant’s environment. For example, sudangrass (and sorghum) contain a chemical, dhurrin, that degrades into hydrogen cyanide, which is a powerful nematicide. (Luna, 1993; Forge, et al, 1995; Wider and Abawi, 2000) Some cover crops have exhibited nematode-suppressive characteristics equivalent to aldicarb, a synthetic chemical pesticide. (Grossman, 1990)
Farmers in Alabama have added sesame into rotation with cotton, peanuts, and soybeans. Nematode levels are reduced and yields significantly increased among those crops in fields previously planted in sesame. Sesame yields averaged 1500 lbs per acre, well above the world average of 500 to 600 lbs per acre. (Anon, 1997a) Research shows that sesame may be an effective rotation crop to control peanut root knot nematode (Meloidogyne arenaria) and southern root knot nematode (Meloidogyne incognita). Sesame rotation is not effective, however, for the Javanese root knot nematode (Meloidogyne javanica). (Starr and Black, 1995) Commercial nematode control products derived from sesame include Dragonfire™ (oil), Ontrol™ (seed meal)—both manufactured by Poulenger USA—and Nemastop™ (ground up sesame plant) from Natural Organic Products.
In South Texas, soybean varieties were shown as possible alternatives to grain sorghum in cotton cropping sequences. Eighteen soybean varieties of maturity group 5, 6, 7, and 8 were tested in Rotylenchulus reniformis-infested soil, either nonfumigated or fumigated with 1,3- dichloropropene. Reproductive rates of R. reniformis were compared in the first year. Both experiments were planted with cotton in the second year to measure the rotational effects of soybean on cotton yield compared with grain sorghum and fallow. The high-yielding soybean cultivars with potential to suppress reniform nematode were “HY574,” “Padre,” “DP7375RR,” and “NK83-30.” (Westphal and Scott, 2005)
A 2000-2002 Maryland study evaluated crop rotations and other cultural practices to manage southern root-knot nematodes and lesion nematodes. Researchers grew nematode-susceptible potatoes and cucumbers, and compared the effect of several summer rotations on nematode problems. A summer rotation of sorghum sudangrass (Sorghum bicolor x Sorghum arundinaceum var. sudanense) reduced the root knot nematode population as effectively as the control treatment (soyabean cultivar with no known root-knot resistance and one nematicide application). Poultry litter/tillage (Year 1) and fallow (Year 2) were equally effective in managing the lesion nematode population. To maintain the effect, the rotations had to be included annually. Either summer or early-autumn sampling dates were more effective than midspring to identify threshold levels of the pests. (Kratochvil et al., 2004)
Nematodes and pH
Cyst nematodes do not hatch well in very acid soils (pH 4) or alkaline soils (pH 8). They do best in soil with a near-neutral pH of 6. This can be used to some advantage. For example, potatoes may be safest from nematode damage in an acid soil, while cabbage and beets can be planted in alkaline soil. But most plants do best at the pH that favors nematodes. |
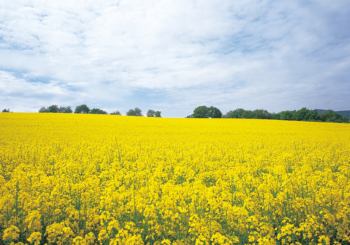
Mustard. Photo: USDA ARS
Researchers have observed that brassicas (e.g., rapeseed, mustard) have a nematode-supressive effect that benefits the following crop in a rotation. This “mustard effect” is attributed to glucosinolate compounds contained in brassica residues. Toxicity is attributed to enzymatically induced breakdown products of glucosinolates, a large class of compounds known as isothiocyanates and nitriles that suppress nematodes by interfering with their reproductive cycle. These glucosinolate breakdown products are similar to the chemical fumigant VAPAM® (metam sodium), which degrades in soil to methyl isothiocyanate. Glucosinolate compounds are also responsible for the pungent flavors and odors of mustards and horseradish. (Brown and Morra, 1997) Jack Brown, PhD, a plant breeder specializing in brassicas at the University of Idaho, has released two biofumigant varieties, “Humus” rapeseed and “IdaGold” mustard, each containing elevated levels of glucosinolates. Cover crop seed for mustards, rapeseed, and oilseed radish are available from a variety of sources. Several Extension Service bulletins describe the use of brassica cover crops in greater detail.
Allelopathic Cover Crops
Some plants produce allelochemicals that function as nematode-antagonistic compounds, such as polythienyls, glucosinolates, cyanogenic glycosides, alkaloids, lipids, terpenoids, steroids, triterpenoids, and phenolics, among compounds from these plants—e.g., castor bean, chrysanthemum, partridge pea, velvetbean, sesame, jackbean, crotalaria, sorghum-sudan, indigo, tephrosia—are exuded during the growing season or released during green manure decomposition. Sunn hemp, a tropical legume, and sorghum-sudan, a prolific grass plant grown for its biomass, are popular nematode-suppressive cover crops that produce the allelochemicals known as monocrotaline and dhurrin, respectively. (Chitwood, 2002; Grossman, 1988; Hackney and Dickerson, 1975; Quarles, 1993; Wang et al., 2002; Williams and Williams, 1990a, 1990b, 1993) |
Here are some examples of how brassica crops are being used to manage nematodes:
- Oil radish as a green manure has dramatically reduced stubby root nematode (Trichodorus) and root lesion nematode (Pratylenchus) in Idaho potato fields. (Anon, 2001)
- Oil radish is used as a “trap crop” for the sugarbeet cyst nematode, its roots exude chemicals that stimulate hatching of nematode eggs. The larvae that emerge are unable to develop into reproductive females—reducing the population densities for the following crop. (Hafez, 1998)
- Rape or mustard plantings in rotation with strawberries have checked the increase of some nematodes. (Brown and Morra, 1997)
- Rapeseed and sudangrass green manures grown prior to potatoes at Prosser, Washington, provided between 72 and 86 percent control of the root-knot nematode in that crop. (Stark, 1995) In the same study, on-farm research in western Idaho showed that rapeseed green manures decreased soil populations of rootlesion nematodes to a greater extent than did sudangrass green manures. Fall sudangrass should be plowed down after it is stressed (i.e., the first frost, stopping irrigation). Winter rapeseed and canola should be incorporated in very early spring. (Cardwell and Ingham, 1996)
Rotation
The best rotation to control the Columbia root-knot nematode in potatoes involves planting a summer non-host crop, followed by a winter cover crop (rapeseed) incorporated as a green manure. Non-host crops include supersweet corn (Crisp and Sweet 710/711), pepper, lima bean, turnip, cowpea, muskmelon, watermelon, squash, rapeseed, canola, mustard, and sudangrass (Trudan 8, Sordan 79). (Ingham, 1990) For root lesion nematode control on potatoes, researchers found that forage pearl millet (Canadian Hybrid 101) and marigold (Crakerjack) as rotation crops with potatoes resulted in fewer root lesion nematodes and increased potato yields than rotation with rye. (Ball-Coelho et al., 2003) |
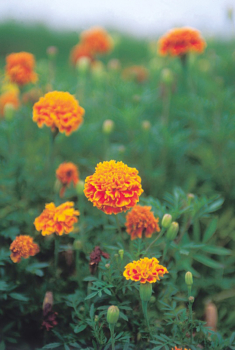
Marigolds. Photo: Clipart.com
Marigold (Tagetes species) is one of the most highly studied crops for its ability to suppress nematodes with antagonistic phytochemical exudates, namely the polythienyls. Research also demonstrates that rhizobacteria living in association with marigold roots are suppressive to root lesion and other nematodes. These multiple effect nematode-control properties can benefit other crops when marigolds are grown in rotation. (Sturz and Kimpinski, 2004) African marigold (Tagetes erecta) and French marigold (Tagetes patula) are popular ornamentals in the horticultural trade with several nematode-suppressive varieties each. (Dover et al., 2003) Muster John Henry or little marigold (Tagetes minuta) is sold as the “Nematicidal” marigold, but it controls a relatively limited range of nematode species and readers should note that it is classified as a noxious weed in California. Tomatoes planted two weeks after African marigolds (Tagetes erecta) were disked into the soil showed a 99 percent reduction in root-lesion nematode damage compared to a tomato-tomato or fallow-tomato rotation. (Grossman, 1999) The French marigold cultivar “Single Gold” provided 99 percent control of nematodes in Dutch tests. (Ogden, 1997) Burpee Seed Co. has carried a French marigold variety known as “Nema-gone.” The most effective marigold cultivars are those that germinate quickly, grow vigorously, and have deep root penetration. Cover crops exhibit tremendous variability in their susceptibility to or suppression of the four major types of plant-parasitic nematodes. For example, cover crops that suppress root-knot nematodes may be susceptible to sting nematodes. It is important to identify the nematode species in the field—and know what their plant hosts and antagonists are—before planning a cover-cropping strategy.
Allies from the Prairie
In Ontario, certain prairie species have been found to provide excellent nematode control when used as a cover crop, including blackeyed susan, gaillardia, and switchgrass, according to Marvin Pritts, PhD, of Cornell University. (Anon, 1996) Another North American native known as “Indian Blanket” or “Blanket Flower” (Gaillardia pulchella) was effective in controlling southern root knot nematode (Meloidogyne incognita) on sweet potato. Tissue extracts of Indian Blanket were lethal to various plant-parasitic nematodes but were innocuous to free-living nematodes. Root exudates of Indian Blanket were lethal to mobile juvenals of M. incognita and were inhibitory to the hatch of eggs at concentrations of 250 parts per million or higher. Indian Blanket could be used to manage southern root knot nematode as a rotation crop, a co-planted crop, or a soil amendment to control root-knot nematode. (Tsay et al., 2004) |
Fields left fallow but kept weed-free for one to two years usually have an 80 to 90 percent per-year reduction in root-knot populations. (Sasser, 1990) This host-free period can be achieved in one season, rather than two years, by disking every ten days all summer. While such disking offers the added advantage to reduce perennial weeds, it is expensive in terms of fuel costs, possible erosion, and loss of organic matter through oxidation. (Ingham, 1996)
Botanical Nematicides
Certain plants are able to kill or repel pests, disrupt their lifecycle, or discourage them from feeding. Some of these—marigolds, sesame, castorbean, and various brassicas—have been discussed previously as nematode-suppressive cover crops. In this section we will look at plants whose extracts or essential oils can be applied as nematicides.
Botanical Nematicides
|
Producers or Distributors
|
Beneficial Nematodes
Steinernema species |
Nitron Industries, Johnny’s Selected Seeds, BioLogic, Hydro-Gardens
|
Biocontrol Bacteria
Deny, Blue Circle (Burkholderia cepacia) Activate (Bacillus chitinosporus) |
Stine Microbial Products
Rincon Vitova |
Biocontrol Fungi
DiTera (Myrothecium verrucaria) MeloCon, BioAct (Paecilomyces lilacinus) |
Valent USA, Peaceful Valley,
Prophyta |
Chitin
ClandoSan Shrimp Shell meal |
Igene Biotech, ARBICO, Peaceful Valley
|
Botanical Nematacide
Nemastop (Organic extracts w/Fatty acids) Dragonfire (sesame oil) Ontrol (sesame meal) Nemagard (ground up sesame plant) Neem cake Armorex (sesame oil, garlic, rosemary eugenol, white pepper) |
Soils Technology Corp
Poulenger USA Poulenger USA Natural Organic Products Monsoon, Peaceful Valley Soils Technology Corp |
Adapted from Quarles, William. 2005. Directory of least toxic pest control products. The IPM Practitioner, Vol. 26, No. 11/12. p. 17.
|
Nitron Industries Inc.
P.O. Box 1447
Fayetteville, AR 72702
800-835-0123
Johnny’s Selected Seeds
184 Foss Hill Rd.
Albion, ME 04910
207-437-4301
BioLogic
P.O. Box 177
Willow Hill, PA 17271
717-349-2789
Hydro-Gardens, Inc.
P.O. Box 25845
Colorado Springs, CO 80936
800-634-6362
Stine Microbial Products
2225 Laredo Trail
Adel, IA 50003
515-677-2605
Rincon Vitova Inc.
P.O. Box 1555
Ventura, CA 93002
800-248-2847
Valent USA
P.O. Box 8025
Walnut Creek, CA 94596
800-624-6094
Peaceful Valley Farm Supply
P.O. Box 2209
Grass Valley, CA 95945
888-784-1722
Prophyta
Inselstrasse 12
D 23999 Malchow
Poel, Germany
Igene (PMG) Biotechology
9110 Red Branch Rd.
Columbia, MD 21045
410-997-2599
ARBICO
P.O. Box 8910
Tucson AZ 85738
800-827-2847
Monsoon Neem Products
P.O. Box 4558
Petaluma, CA 94955
707-778-6137
Soils Technology Corp
2103 185th St.
Fairfield, IA 52556
800-221-7645
Poulenger USA
3705 Century Blvd. #3
Lakeland, FL 33811
866-709-8102
Natural Organic Products
7105 Rossiter St.
Mt. Dora, FL 32757
325-383-8252
For hundreds of years, Indian farmers have used the neem tree (Azadirachta indica) for its pesticidal, antifungal, and antifeedant properties. In research trials, potting soil amended with plant parts from the neem tree and Chinaberry tree (Melia azadirach) inhibited root-knot nematode development on tomatoes. (Siddiqui and Alam, 2001) However, no neem products are currently registered in the U.S. for use against nematodes. Margosan-O™, Azatin™, Superneem 4.5™, Neemix™, and Triact™ are neem products registered as insecticides, fungicides, and miticides. Neem cake, made from crushed neem seeds, provides nitrogen in a slow-release form in addition to protecting plants against parasitic nematodes. It is sold as a fertilizer in the U.S. through many farm and garden supply stores. Neem cake can be mixed with fertilizers such as composted manures, seaweed, and kelp. Recommended rates are 180 to 360 lbs. per acre or 2 lbs. per 100 to 160 sq. ft. (Anon, 1998) Neem cake is toxic to plant-parasitic nematodes and is not as detrimental to beneficial free-living soil organisms. (Riga and Lazarovits, 2001) In greenhouse trials, 1 percent neem cake (mass/mass soil) caused a 67 to 90 percent reduction in the number of lesion (Pratylenchus penetrans) and root-knot (Meloidogyne hapla) nematodes in tomato roots grown in three different soils. In the field, 1 percent neem cake (mass/mass soil) reduced the number of lesion nematodes by 23 percent in corn roots and 70 percent in soil around roots. (Abbasi et al., 2005)
Essential oils from various plants have shown promise as potential sources for new nematicides. Most of these plants are aromatic and culinary herbs that contain the nematicidal compounds carvacrol and thymol. At very low concentrations (1000 micrograms per liter, or .001 gm per liter, or .0038 gm per gal, or 0.38 gm per 100 gal) several oils immobilized juvenile root-knot nematodes and some also reduced hatching of eggs. The essential oils from the following plants ranked the highest for nematicidal activity: caraway, fennel, applemint, spearmint, Syrian oregano, and oregano. (Oka et al., 2000) The toxicity of the essential oil from wormwood or Sweet Annie (Artemisia annua) leaves was evaluated in vitro against second-stage juveniles (J2) of the root knot nematode (Meloidogyne incognita) and pre-adults of the reniform nematode (Rotylenchulus reniformis). Complete mortality (100 percent) of both nematodes was found in 500 and 250 parts per million concentrations of the essential oil and gradually decreased with lower concentrations. (Shakil et al., 2004)
Biocontrols
Several microbial pathogens have been developed into commercial formulations against nematodes. These include the bacteria Pasteuria penetrans (formerly known as Bacillus penetrans), Bacillus thuringiensis (available in insecticidal formulations) and Burkholderia cepacia. Nematicidal fungi include Trichoderma harzianum, Hirsutella rhossiliensis, Hirsutella minnesotensis, Verticillium chlamydosporum, Arthrobotrys dactyloides, and Paceilomyces lilacinus. Another fungus, Myrothecium verrucaria, found to be highly effective in the control of nematodes (Anon, 1997b), is available in a commercial formulation, DiTera™, from Abbott Laboratories. Circle One, Inc. offers a combination of several mycorrhizal fungal spores in a nematode-control product called Prosper-Nema™. Stein Microbial products offers the bacterium Burkholderia cepacia in a product called Deny™ and Blue Circle™. Rincon-Vitova offers a product called Activate™ whose active ingredient is the bacterium Bacillus chitinosporus. (Quarles, 2005)
The insect-attacking nematode Steinernema riobravis can provide root-knot nematode control comparable to that achieved with chemical nematicides. (Grossman, 1997) Although the exact mechanisms of control are not known, researchers hypothesize that an allelochemical is involved (perhaps manufactured by symbiotic bacteria that live within S. riobravis) that repels plantparasitic nematodes. Recent research measured the effect of beneficial nematodes on root-knot nematodes (Meloidogyne species) infecting tomatoes and peanuts. In the laboratory, peanut seedlings treated with the beneficial nematodes Steinernema feltiae and Steinernema riobrave showed resistance to pest nematodes. In the greenhouse, scientists tested application levels and timing on peanut and tomato plants. On peanuts, pre- and post-infestation applications of S. feltiae suppressed M. hapla penetration but not egg production. Only pre-infestation applications of S. riobrave suppressed M. hapla. The tomatoes were infested with Meloidogyne incognita eggs and treated with Steinernema glaseri or Heterorhabditis megidis applied at the same times as the tomato treatments. The low rate of S. glaseri suppressed M. incognita penetration into tomato roots and the high rate of S. glaseri reduced egg production. (Pérez and Lewis, 2004) Those interested in using this biocontrol will need to experiment with application rates and techniques to develop methods best suited to their operations. Additional information on insect parasitic nematodes can be found on an Ohio State University website.
A soil-dwelling predatory mite, Hypoaspis miles, preys primarily on fungus-gnat larvae but will also attack spring tails, thrips, and nematodes. (Anon, No date) These mites are available commercially for the control of fungus gnats in greenhouse production of tomatoes, peppers, cucumbers, flowers, and foliage plants. The mites are applied to the planting media.
It is clear that a wide range of organisms feed on, kill, or repel nematodes. These organisms are most effective, and are found most commonly, in healthy, well-managed soils.
Plant Resistance
Generally speaking, a resistant cultivar is more effective against sedentary endoparasitic species such as root-knot and cyst nematodes than against “grazing” ectoparasitic species. Root-knot and cyst nematodes spend most of their lifecycle within the root, relying on specialized cells for feeding. Upon entering the roots of resistant cultivars, these nematodes become trapped as the feeding cells necessary for their survival fail to develop.
Many crop cultivars—tomatoes and soybeans in particular—have been specifically bred for nematode resistance. The “N” designation on tomato seed packages (usually as part of “VFN”) refers to nematode resistance. A few cultivars of potatoes are resistant to the golden nematode, which is a pest only in a small area of the northeastern U.S. Although most cultivars of potatoes are susceptible to infection by nematodes, some varieties tolerate infection better than others. For example, population densities of root-lesion nematodes (Pratylenchus penetrans) that would affect yield in “Superior” are tolerated with little effect by “Russet Burbank.” (MacGuidwin, 1993)
Richard L. Fery, PhD, a geneticist at the USDA’s Agricultural Re search Service in Charleston, South Carolina, developed two nematode-resistant varieties of bell pepper, “Charleston Belle” and “Carolina Wonder,” available from commercial seed companies. (Sanchez, 1997) Charleston Belle and its susceptible parent, “Keystone Resistant Giant,” were compared as spring crops to manage the southern root-knot nematode (Meloidogyne incognita) in autumn-cropped cucumber and squash. Cucumber grown in plots following Charleston Belle had lower root gall severity indices than in crops following Keystone Resistant Giant. Cucumber yields were 87 percent heavier and numbers of fruit 85 percent higher in plots previously planted to Charleston Belle than to Keystone Resistant Giant. Squash grown in plots following Charleston Belle had lower root gall severity indices than those following Keystone Resistant Giant. Squash yields were 55 percent heavier and numbers of fruit 50 percent higher in plots previously planted to Charleston Belle than to Keystone Resistant Giant.
These results demonstrate that root-knot nematode-resistant bell pepper cultivars such as Charleston Belle are useful tools to manage M. incognita in double-cropping systems with cucurbit crops. (Thies et al., 2004) Nematode-tolerant or resistant cultivars of snap beans (“Harvester” and “Alabama #1”), lima beans (“Nemagreen”), and sweet potatoes (“Carolina Bunch,” “Excel,” “Jewel,” “Regal,” “Nugget,” and “Carver”) also exist and may be used in a similar strategy to reduce nematode levels for crops that follow.
The choice of nematode-resistant rootstock for perennial fruit production is important to ensure protection of trees and vines against these unseen pests. Consult with a local farm advisor to confirm that the rootstock you choose is appropriate for the area.
Table 1. Nematode-resistant rootstock for perennial fruit.
|
|
Fruit
|
Rootstock
|
Apple
|
No commonly used rootstock is completely resistant (Ohlendorf, 1999)
|
Pears
|
Bartlett, Quince (slight resistance) (Ohlendorf, 1999)
|
Asian Pear
|
Calleryana (Anon, 2002)
|
Citrus
|
Poncirus trifoliate, lime, rough lemon, sour orange (Inserra et al., 1994) Forner-Alcaide 5 (Forner et al., 2003)
|
Grapes
|
Freedom, Harmony, Dog Ridge, Ramsey (Cousins, 1997) VR039-16 (McHenry et al., 2004)
|
Peach & Nectarines
|
Nemaguard, Nemared, Citation, Hansen 536 (Anon, 2004)
|
Plums
|
Myrobalan 29-C, Marianna 2624 (Anon, 2004)
|
Apricots & Almonds
|
Nemaguard, Nemared, Myrobalan, Marianna 2624 (Anon, 2004)
|
Cherries
|
Mazzard, Mahaleb (Anon, 2004)
|
Breeding for nematode resistance in most crops is complicated by the ability of the nematode species (primarily cyst nematodes and root-knot nematodes) to develop races or biotypes that overcome the genetic resistance factors in the crop. In order to maintain resistant crop cultivars on farms, researchers suggest that susceptible and resistant cultivars be planted in rotation. When a nematode-resistant cultivar is planted, nematode populations generally decrease, but over the course of the growing season the few nematodes in a particular population capable of overcoming this resistance begin to increase. If in the following season the farmer plants a susceptible cultivar, the overall nematode numbers will still be low enough to avoid significant yield reduction, but more importantly, the selective pressure favoring the increase of the “counter-resistant” bio types is removed. As long as the farmer continues to alternate susceptible and resistant cultivars (and, better yet, incorporate non-host crops into the rotation), the nematodes can be kept at non-damaging levels.
Transgenic crop resistance to nematodes and other pests is being developed for numerous crops by various companies worldwide. The use of genetically modified organisms is not accepted in organic production systems.
Red Plastic Mulch
Springtime field tests at the Agricultural Research Service in Florence, South Carolina, indicate that red plastic mulch suppresses root-knot nematode damage in tomatoes. According to Michael Kasperbauer, one of the researchers, “The red mulch reflects wavelengths of light that cause the plant to keep more growth above ground, which results in greater yield. Meanwhile, the plant is putting less energy into its root system—the very food the nematodes feed on. So reflection from the red mulch, in effect, tugs food away from the nematodes that are trying to draw nutrients from the roots.” The research team planted tomatoes in sterilized soil, mulched them with red or black plastic, and inoculated the roots with nematodes. Plants inoculated with 200,000 nematode eggs and mulched with black plastic produced 8 pounds of tomatoes, while those mulched with red plastic produced 17 pounds. The red mulch is available commercially from Ken-Bar, Inc., of Reading, Massachusetts.
Solarization
Soil solarization, a method of pasteurization, can effectively suppress most species of nematode. However, it is consistently effective only where summers are predictably sunny and warm. The basic technique entails laying clear plastic over tilled, moistened soil for approximately six to eight weeks. Solar heat is trapped by the plastic, raising the soil temperature. The incorporation of poultry litter prior to solarization, or use of a second layer of clear plastic, can reduce effective solarization time to 30 days. (Brown et al., 1989; Stevens et al., 1990) Brassica residues are also known to increase the solarization effect, in a process known as biofumigation. The plastic holds in the gaseous breakdown products of the brassica crop (or food processing wastes), thereby increasing the fumigation-like effect. (Gamliel and Stapleton, 1993) Large-scale field experiments using cabbage residues with solarization obtained results comparable to solarization combined with methyl bromide. (Chellami et al., 1997)
Solarization is well documented as an appropriate technology for control of soilborne pathogens and nematodes, but the economics of purchasing and applying plastic restrict its use to high-value crops. Further information on solarization is available from ATTRA on request.
Soil Steaming
Steaming the soil suppresses nematodes in a manner similar to solarization. There are prototype steam machines capable of performing field applications, but steaming is probably economical only for greenhouse operations or small plantings of high-value crops. (Grossman and Liebman, 1995) For more information on steaming, contact ATTRA. |
Flooding
In certain parts of the country (e.g., Tule Lake in California) where water is usually available and water pumping equipment and dikes already exist, and for certain large-scale monocultures (e.g., potatoes), flooding is sometimes used as a management tool to control nematodes. But for most farms, it is probably not an option. Flooding the soil for seven to nine months kills nematodes by reducing the amount of oxygen available for respiration and increasing concentrations of naturally occurring substances—such as organic acids, methane, and hydrogen sulfide—that are toxic to nematodes. (MacGuidwin, 1993) However, it may take two years to kill all the nematode egg masses. (Yepsen, 1984) Flooding works best if both soil and air temperatures remain warm. An alternative to continuous flooding is several cycles of flooding (minimum two weeks) alternating with drying and disking. (MacGuidwin, 1993) But note that insufficient or poorly managed flooding can make matters worse, as water is also an excellent means of nematode dispersal.
Summary
Each combination of nematode and host is different. As the nematode population density reaches a certain level, the host crop yield suffers. Some hosts support faster population increases than others. Environmental conditions can also affect the relative dangers posed by nematode populations. (Dropkin, 1980) As we begin to develop a better understanding of the complex ecologies of soils and agricultural ecosystems, more strategies for cultural and biological control of nematodes will be developed. The trick will be fine-tuning these general strategies to the unique ecology, equipment, and financial situation of each farm.
References
Abbasi, P.A.; E. Riga, K.L. Conn, and G. Lazarovits. 2005. Effect of neem cake soil amendment on reduction of damping-off severity and population densities of plant-parasitic nematodes and soilborne plant pathogens. Canadian Journal of Plant Pathology. Vol. 27, No. 1, pp. 38-45.
Akhtar, A., and A. Malik. 2000. Roles of organic soil amendments and soil organisms in the biological control of plant parasitic nematodes: a review. Bioresource Technology. 74. p 35.
Akhtar, M., and M. Mashkoor Alam. 1993. Utilization of waste materials in nematode control: a review. Bioresource Technology. Vol. 45.. p. 1—7.
Anon. 1996. Prairie species control nematodes. The Great Lakes Vegetable Growers News. February. p. 33.
Anon. 1997a. Sesame rotation controls nematodes and provides Alabama a new cash crop. Highlights of Agricultural Research. Vol. 44, No. 1, Spring 1997. Downloaded May 2005.
Anon. 1997b. DiTera: Controlling nematodes biologically. Methyl Bromide Alternatives. January. p. 8—9.
Anon. 1998. Plasma Neem Cake. Plasma Power Web site. Downloaded April 2002.
Anon. 2001. Oil radish green manure continues promise against nematodes. The Grower. June—July. p. 7.
Anon. 2002. Burchell Nursery Inc. Web site. Downloaded May 2005.
Anon. 2004. Rootstock description. Bay Laurel Nursery Web Page. Downloaded May 2004.
Anon. No date. Hypoaspis miles, Agrobiologicals product page. Downloaded June 2002.
Ball-Coelho, B.; A. J. Bruin; R. C. Roy; E. Riga. 2003. Forage pearl millet and marigold as rotation crops for biological control of root-lesion nematodes in potato. Agronomy Journal, Vol. 95, No. 2. p. 282-292.
Barker, K.R., and S.R. Koenning. 1998. Developing sustainable systems for nematode management. Annual Review of Phytopathology. Vol. 36. p. 165-205.
Brown, J.E. , M.G. Patterson, and M.C. Osborn. 1989. Effects of clear plastic solarization and chicken manure on weed control. p. 76—79. In: Proceedings of the 21st National Agricultural Plastics Congress. Nat. Ag. Plastics Assoc., Peoria, IL.
Brown, Paul D., and Matthew J. Morra. 1997. Control of soil-borne plant pests using glucosinolate-containing plants. p. 167—215. In: Donald L. Sparks (ed.) Advances in Agronomy. Vol. 61. Academic Press, San Diego, CA.
Cardwell, Derek, and Russ Ingham. 1996. Management of practices to suppress Columbia root-knot nematode. Pacific Northwest Sustainable Agriculture. October p. 6.
Chellami, D.O., S.M. Olson, D. J. Mitchell, I. Secker, and R. McSorley. 1997. Adaptation of soil solarization to the integrated management of soilborne pests of tomato under humid conditions. Phytopathology. Vol. 87,. No. 3. pp. 250—258.
Chitwood, David J. 2002. Phytochemical based strategies for nematode control. Annual Review of Phytopathology. Vol. 40. p. 221—249.
Cousins, Peter. 1997. Root-knot nematode resistance in grape rootstocks. Dept. of Viticulture and Enology, University of California.
Dover, K. E., R. McSorley, K., H. Wang. 2003. Marigolds as Cover Crops. Department of Entomology & Nematology, University of Florida. Downloaded November 2005.
Dropkin, Victor H. 1980. Introduction to Plant Nematology. John Wiley & Sons, New York, NY. p. 38—44, 242—246, 256.
Dunn, Robert A. 1995. Diagnosing Nematode Problems. Florida Agricultural Information Retrieval System (FAIRS) . Document RF-NG006, Department of Entomology and Nematology, Florida Cooperative Extension Service, Institute of Food and Agricultural Sciences, University of Florida. Reviewed: April 1995.
Fiola, J., and N. Lalancettle. 2000. 2000 New Jersey Commercial Strawberry Pest Control Recommendation I. P. 2. In: Rutgers Cooperative Extension Bulletin FS193.
Forge, Thomas A., Russell E. Ingham, and Diane Kaufman. 1995. Winter cover crops for managing root-lesion nematodes affecting small fruit crops in the Pacific Northwest. Pacific Northwest Sustainable Agriculture. March. p. 3.
Forner, J. B., M. A. Forner-Giner, and A. Alcaide. 2003. Forner-Alcaide 5 and Forner-Alcaide 13: two new citrus rootstocks released in Spain. HortScience, Vol. 38, No. 4. pp. 629-630.
Gamliel, A., and J.J. Stapleton. 1993. Characterization of antifungal volatile compounds evolved from solarized soil amended with cabbage residues. Phytopathology. Sept. p. 899—905.
Grossman, Joel. 1988. Research notes: New directions in nematode control. The IPM Practitioner. February. p. 1—4.
Grossman, Joel. 1990. New crop rotations foil root-knot nematodes. Common Sense Pest Control. Winter. p. 6.
Grossman, Joel. 1997. Root-knot nematode biocontrol. The IPM Practitioner. April. p. 15.
Grossman, Joel. 1999. ESA and APS joint meeting—part 8. IPM Practitioner. October. p. 13.
Grossman, Joel, and Jamie Liebman. 1995. Alternatives to methyl bromide—steam and solarization in nursery crops. The IPM Practitioner. July. p. 3.
Hackney, R.W., and O.J. Dickerson. 1975. Marigold, castor bean, and chrysanthemum as controls of Meloidogyne incognita and Pratylenchus alleni. Journal of Nematology. Vol. 7, No. 1. p. 84—90.
Hafez, Saad L. 1998. Management of Sugarbeet Cyst Nematode. University of Idaho Cooperative Extension. CIS 1071. p. 2.
Horst, Kenneth R. 1990. Westcott’s Plant Disease Handbook. 5th ed. Van Nostrand Reinhold, New York, NY. p. 306—307.
Ingham, R.E. 1990. Biology and control of root-knot nematodes of potato—Research report. Proceedings of the Oregon Potato Conference and Trade Show. p. 109—120, 18—36.
Ingham, Elaine. 1996. The Soil FoodWeb: Its Importance in Ecosystem Health. 13 p.
Inserra, R.N., L.W. Duncan, J.H. O’Bannon, and S.A. Fuller. 1994. Citrus nematode biotypes and resistant citrus rootstocks in Florida. University of Florida Cooperative Extension Service. Accessed June 2002.
Kodira, U.C., and B.B. Westerdahl. 1995. Potato Pest Management Guidelines. UC Statewide IPM. University of California, Davis, CA. 3 p.
Kratochvil, R. J.; S. Sardanelli; K. Everts; E.Gallagher. 2004. Evaluation of crop rotation and other cultural practices for management of root-knot and lesion nematodes. Agronomy Journal, Vol. 96, No. 5. pp. 1419-1428.
Luna, J. 1993. Crop rotation and cover crops suppress nematodes in potatoes. Pacific Northwest Sustainable Agriculture. March. p. 4—5.
MacGuidwin, A.E. 1993. Management of Nematodes. p. 159—166. In: Randell C. Rowe (ed.) Potato Health Management. APS Press, St. Paul, MN.
McHenry, M. V.; D. Luvisi; S. A. Anwar; P. Schrader; S. Kaku. 2004. Eight-year nematode study from uniformly designed rootstock trials in fifteen table grape vineyards. American Journal of Enology and Viticulture, Vol. 55, No. 3. pp. 218-227.
Ogden, Shepherd. 1997. Marigolds bite back. National Gardening. March—April. p. 21.
Ohlendorf, Barbara L.P. 1999. Integrated pest management for apples and pears. University of California Publication No. 3340. p. 198.
Oka, Y., S. Nacar, E. Putieusky, U. Ravid, Y. Zohara, and Y. Spiegal. 2000. Nematicidal activity of essential oils and their components against the root knot nematode. Phytopathology 90 (7). p. 710—715.
Oka, Y., and U. Yermiyahu. 2002. Suppressive effects of composts against the root-knot nematode Meloidogyne javanica on tomato. Nematology, Vol. 4, No. 8, pp. 891-898.
Peet, Mary. 1996. Sustainable Practices for Vegetable Production in the South. Focus Publishing, Newburyport, MA. p. 75—77.
Pérez, E. E. and E. E. Lewis. 2004. Suppression of Meloidogyne incognita and Meloidogyne hapla with entomopathogenic nematodes on greenhouse peanuts and tomatoes. Biological Control, 2004, Vol. 30, No. 2. pp. 336-341.
Ploeg, Antoon. 2001. When nematodes attack is important. California Grower. October. p. 12-13.
Quarles, William. 1993. Rapeseed green manure controls nematodes. The IPM Practitioner. April. p. 15.
Quarles, William. (ed.). 2005. 2005 directory of least toxic pest control products. The IPM Practitioner, Vol. 26, No. 11/12. p. 17.
Riga, E., and G. Lazarovits. 2001. Development of an organic pesticide based on neem tree products. American Phytopathological Society/ Mycological Society of America/ Society of Nematology Joint Meeting Abstracts of Presentations. Salt Lake City, Utah. Phytopatology 91: S141. Publication no.P2001-0096-SON.
Sasser, J. N. 1990. Plant-parasitic Nematodes: The Farmer’s Hidden Enemy. North Carolina State University Press, Raleigh, NC. p. 47—48.
Sanchez, Pat. 1997. For pepper growers, built-in nematode resistance. Agricultural Research. October. p. 12—13.
Shakil, N. A.; D. Prasad; D. B. Saxena; A. K Gupta. 2004. Nematicidal activity of essential oils of Artemisia annua against root-knot and reniform nematodes. Annals of Plant Protection Sciences, Vol. 12, No. 2. pp. 403-408.
Siddiqui, M.A. and M.M. Alam. 2001. The IPM Practioner. April. p. 9—11.
Stark, J.C. 1995. Development of Sustainable Potato Production Systems for the Pacific Northwest. SARE Final Report.
Starr J. L. and M. C. Black. 1995. Reproduction of Meloidogyne arenaria, M. incognita, and M. ]avanica on Sesame. Supplement to the Journal of Nematology 27(4S):624-627.
Stevens, C., V.A. Khan, and A.Y. Tang. 1990. Solar heating of soil with double plastic layers: a potential method of pest control. p. 163—68. In: Proceedings of the 22nd National Agricultural Plastics Congress. Nat. Ag. Plastics Assoc., Peoria, IL.
Stirling, G.R. 1991. Biological Control of Plant Parasitic Nematodes. CAB International, Wallingford, UK. 275 p.
Sturz, A. V. and J. Kimpinski. 2004. Endoroot bacteria derived from marigolds (Tagetes species) can decrease soil population densities of root-lesion nematodes in the potato root zone. Plant and Soil. Vol. 262, No. 1-2. pp. 241-249.
Thies, J. A.; R. F. Davis; J. D. Mueller; R. L Fery; D. B. Langston; G. Miller. 2004. Double-cropping cucumbers and squash after resistant bell pepper for root-knot nematode management. Plant Disease, 2004. Vol. 88, No. 6. pp. 589-593.
Tsay, T. T.; S. T. Wu; Y. Y. Lin. 2004. Evaluation of Asteraceae plants for control of Meloidogyne incognita. Journal of Nematology. Vol. 36, No. 1. pp. 36-41.
Walker, G. E. 2004. Effects of Meloidogyne javanica and organic amendments, inorganic fertilisers and nematicides on carrot growth and nematode abundance. Nematologia Mediterranea, Vol. 32, No. 2, pp. 181-188.
Wang, K. H., R. McSorley, R. N. Gallaher. 2004. Effect of Crotalaria juncea amendment on squash infected with Meloidogyne incognita. Journal of Nematology, 2004. Vol. 36, No. 3. pp. 290-296.
Wang, K. H., B. S. Sipes, and D. P. Schmitt. 2002. Suppression of Rotylenchulus reniformis by Crotalaria juncea, Brassica napus, and Target erecta. Nematropica. Vol. 31. p. 237-251.
Westphal, A. and A. W. Scott Jr. 2005. Implementation of soybean in cotton cropping sequences for management of reniform nematode in South Texas. Crop Science, 2005, Vol. 45, No. 1. pp. 233-239.
Wider, T.L., and G.S. Abawi. 2000. Mechanism of suppression of Meloidogyne hapla and its damage by a green manure of Sudan grass. Plant Disease. Vol. 84. p. 562-568.
Williams, Greg, and Pat Williams (eds.) 1990a. Sesame residues vs. harmful nematodes. HortIdeas. March. p. 35.
Williams, Greg and Pat Williams (eds.) 1990b. (Some) plant nutrients repel harmful nematodes. HortIdeas. June. p. 63.
Williams, Greg, and Pat Williams (eds.) 1993. Wheat vs. nematodes causing peach tree short life. HortIdeas. July. p. 76.
Yepsen, Roger B. Jr. (ed.) 1984. The Encyclopedia of Natural Insect & Disease Control. Rev. ed. Rodale Press, Emmaus, PA. p. 267—271.
Further Resources
Agbenin, N. O., A. M. Emechebe, P. S. Marley. 2004. Evaluation of neem seed powder for Fusarium wilt and Meloidogyne control on tomato. Archives of Phytopathology and Plant Protection, Vol. 37, No. 4. pp. 319-326
Budh Ram, and B. L. Baheti. 2003. Management of reniform nematode, Rotylenchulus reniformis on cowpea through seed treatment with botanicals. Current Nematology, Vol. 14, No1/2. pp. 27-30.
Hagan, A. K, W. S. Gazaway, E. J. Sikora. 1994. Nematode suppressive crops. Circular ANR-856, Alabama A&M and Auburn Universities. Accessed April 2005.
Kiewnick, S, and R. A Sikora. 2004. Optimizing the efficacy of Paecilomyces lilacinus (strain 251) for the control of root-knot nematodes. Communications in Agricultural and Applied Biological Sciences, 2004, Vol. 69, No. 3, pp. 373-380.
Koenning, S. R., Edmisten, K. L., Barker, K. R., Bowman, D. T., and Morrison, D. E. 2003. Effects of rate and time of application of poultry litter on Hoplolaimus columbus on cotton. Plant Dis. 87:1244-1249.
Morris, J. B. and J. T. Walker. 2002. Non-traditional legumes as potential soil amendments for nematode control. Journal of Nematology, 2002, Vol. 34, No. 4. pp. 358-361.
Tiyagi, S. A. and Ajaz Shamim. 2004. Biological control of plant parasitic nematodes associated with chickpea using oil cakes and Paecilomyces lilacinus. Indian Journal of Nematology, Vol. 34, No1, pp. 44-48.
Web Resources
Nematodes in Commercial Vegetables
Michigan State University
Methyl Bromide
U.S. Environmental Protection Agency
The Sting Nematode
Kansas State University
Nematodes: Management Guidelines for Kansas Crops
Kansas State University
Root and Soil Analyses for Nematodes in Corn
University of Nebraska-Lincoln
Sampling for Nematodes in Corn
University of Nebraska-Lincoln
Crop Scouting Manual
University of Wisconsin Extension
The Soybean Cyst Nematode Management Guide
North Central Soybean Research Program
Nematodes: Alternative Controls
By Martin Guerena, NCAT Agriculture Specialist
Published April 2006
© NCAT
IP287
This publication is produced by the National Center for Appropriate Technology through the ATTRA Sustainable Agriculture program, under a cooperative agreement with USDA Rural Development. ATTRA.NCAT.ORG.