Nutrient Cycling in Pastures
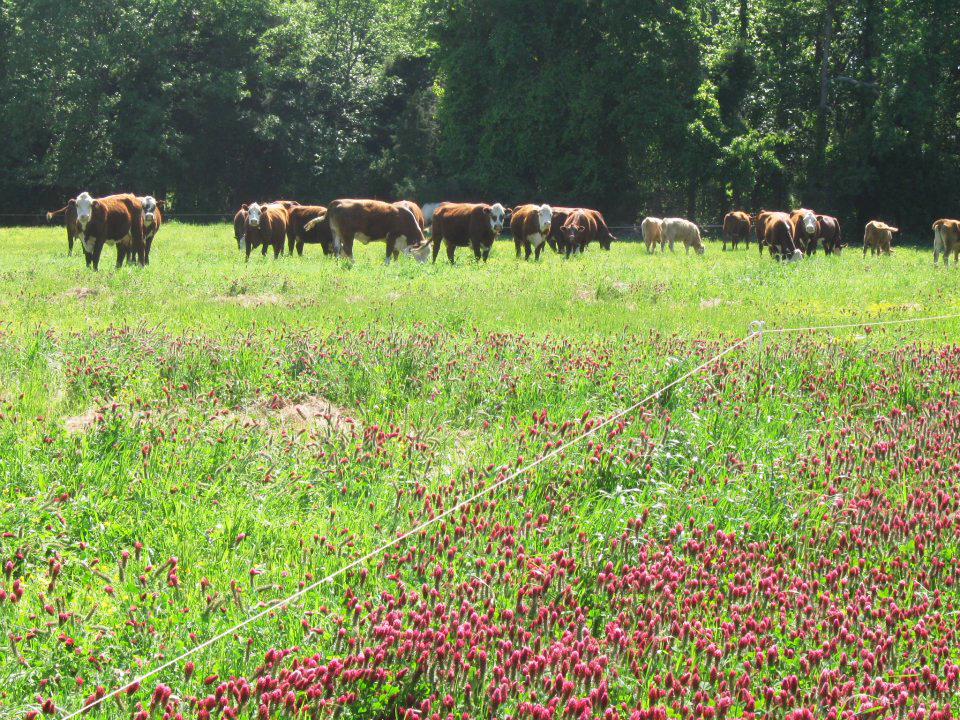
Cattle grazing a diverse array of pasture forage in a rotational grazing system in Arkansas. Photo: Nina Prater, NCAT
By Dave Scott and Nina Prater, NCAT Agriculture Specialists
Abstract
This publication looks at the pathways and drivers that move nutrients into, out of, and within pasture systems. It attempts to provide a clear, holistic understanding of how nutrients cycle through pastures and what the producer can do to enhance the processes to create productive, regenerative, and resilient farm and ranch systems. Effective management of nutrient cycling in pastures is simply understanding how nature cycles nutrients in natural grasslands and then mimicking those processes.
Contents
Introduction
The Rhizosphere
The Photosynthetic and Microbial Bridges
Soil Food Web
Organic Matter
Abiotic Soil Properties
Soil pH
The Base of the Soil Food Web: Bacteria and Fungi
The Predators: Protozoa and Nematodes
Macroorganisms: Earthworms and Dung Beetles
Herbivores’ Role in Cycling Nutrients
Managing for Optimal Pasture Nutrient Cycling
Nutrient Cycling’s Effect on the Water Cycle
Plot Your Progress: Monitor
Conclusion
References
Further Resources
Introduction
Looking out across a pasture, the peacefulness of the scene is deceptive. Above and below the soil surface is an ecosystem full of plants, predators, and prey, enacting the drama of competition for nutrients, survival, and the inevitable eventuality, death and decay. Within the pasture system, nutrients are moving through the food web every second, constantly changing their state, location, and availability. The farmer or rancher acts as the director of this intricate dance, and the management decisions she or he makes will dictate how efficiently the system operates as a whole.
One point we emphasize throughout this publication is how interconnected each nutrient cycle is. Traditional agricultural textbooks typically divide the nutrient cycles by nutrient, forcing weary students to memorize how nitrogen (N) cycles separately from how phosphorus (P) cycles, and don’t forget about the potassium (K) cycle. And what about carbon (C), water, and all those essential micronutrients? In reality, nothing in the pasture environment stays within the bounds of a neat and tidy diagram. Nutrient cycles happen simultaneously and are intertwined with each other. The life cycles of all the different living organisms within and above the soil fuel and drive the movements of all plant nutrients.
Nutrient cycling in pastures is dependent on the photosynthetic and microbial pathways. Light from the sun is photosynthesized into chemical energy, which in turn is exuded out of plant roots in the form of simple sugars and proteins. These compounds feed billions of microbes in the rhizosphere, in exchange for minerals that have been biologically transformed into plant-available nutrients. How we manage our pastures determines the efficiency of this exchange—and our profitability.
The Rhizosphere
One of the first things we as graziers need to fathom is that there is a virtually unlimited source of plant nutrients on earth. As long as there is bedrock, there will be plant nutrients. The catch is how to make them plant-available.
Plant-available nutrient: a nutrient in the chemical form that can be absorbed by a plant. Often these are water-soluble ions, such as nitrate (NO3–), phosphate (H2PO4–), and potassium (K+).
Within the rhizosphere, we can see how plants access these nutrients. The rhizosphere is the region of the soil that is within half an inch of plant roots. This may seem like an insignificant amount of space, but in a functional pasture soil it is a dense, interconnected array of ions, enzymes, membranes, and microbes. Think of it like this: in Montana, a winter sky far from any city has enough stars to light up a moonless night.
So it is in the soil, where, if microbes were stars, the rhizosphere would be the Milky Way, a sparkling band of life. Picture the bacteria and fungi clustering around a root, feasting on root exudates, surrounded by protozoa and nematodes feasting, in turn, on them.
Root exudates are substances that are emitted from plant roots in the form of sugars, proteins, and fatty acids. They are essential for a healthy microbial soil population and a key component of the microbial bridge.
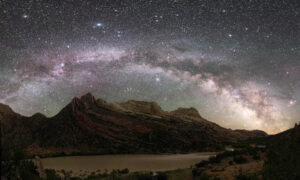
Can you imagine this as a single root under magnification? All of the stars would then be microbes clustered in the rhizosphere surrounding the root. Photo: National Park Service
Pause and consider the fact that there are a million times more bacteria and fungi in the rhizosphere than in the rest of the soil (Ingham, 2014). The rhizosphere is a bustling region of biological activity where fungi and bacteria colonize around sand, silt, and clay particles, engulfing minerals into their cells through enzymatic action. Predation on these bacteria and fungi by protozoa and nematodes releases soluble plant nutrients into the rhizosphere, and the arbuscular mycorrhizal fungi link plant roots with other microbes through their fungal roots to share information via biochemical messaging. These plant-microbe relationships are symbiotic, where plants provide the sugars via photosynthesis, and the microorganisms bring nutrients and water to the plants that would otherwise be inaccessible to them. Every second of every day, every cubic centimeter of rhizosphere is alive with the absorption, predation, elimination, and uptake of every plant nutrient needed. What starts out in bedrock and organic matter, along with water and gases, ends up in several tons of forage dry matter canopy and an equal amount of roots. This occurs in nature without a single trip to the fertilizer co-op.
How do we grow a large amount of pasture forage with no fertilizer? We learn nature’s system, and the way that plants direct soil biology to assist in their continual growth. Let’s look at the key players.
The Photosynthetic and Microbial Bridges
Plants are responsible for life as we know it, using sunlight to bridge the divide between the abiotic physical elements—minerals, water, and gases—and life. Through photosynthesis, plants use energy from sunlight to turn carbon dioxide (CO2) and water (H2O) into atmospheric oxygen (O2) and the most basic carbohydrate, glucose (C6H12O6). In fact, plants get approximately 95% of the elements they need from carbon dioxide and water (Phillips, 2017). Leaves are nature’s solar panels, transforming the energy of the sun into more leaves, stems, roots, flowers, and seeds.
However, it doesn’t stop there. As much as two-thirds of the sugars that plants produce are sent to the roots and are exuded out into the soil, where microorganisms and fungi are waiting to consume them (Phillips, 2017). This isn’t just some kind of inefficient leak in the plants’ systems. It is the foundation for the essential symbiotic relationships plants maintain with their underground partners. This is critical to understand if we want to manage the rhizosphere to ensure that adequate nutrients are available to plants.
The plants send complexes of sugars, proteins, and other compounds into the soil in order to feed the microbes and arbuscular mycorrhizal fungi that will in turn help them access more nutrients and water, which would otherwise be outside the reach of roots and root hairs. This process has come to be called the “liquid carbon pathway,” because it is the way soluble carbon in the form of sugars enters the soil, and it is a significant source of soil organic matter (Jones, 2015). Whereas the bridge of photosynthesis moves energy in one direction—from the sun to the plant—the liquid carbon exchange between plants and soil organisms is a busy, bi-directional thoroughfare shuttling energy, nutrients, and water back and forth. The best part is, this relationship benefits both the organisms above ground (including the grazier), and the organisms in the soil.
Soil Food Web
The liquid carbon pathway is one avenue that brings nutrients into the soil to feed the soil food web, but there are more. Let’s say you’ve been a diligent grazier, and you made sure your livestock left behind a thick thatch of residual, trampled forage after grazing an area for a short period of time.
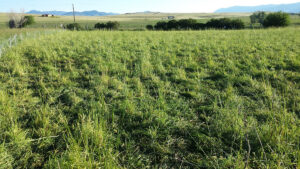
Trampled grass. Seventy thousand live-weight pounds per acre for 24 hours produced this. Look at what is left for soil microbes. Photo: Dave Scott, NCAT
What happens next? Much of that trampled forage will remain on the surface for a period of time, protecting the soil from raindrop impact and wind and also helping to keep soil moisture from evaporating. But after a while, it will start to break down and disappear. Where is it going? If you dig into the soil, you may be able to see some of the living things that are using this residue as their foodstuff, turning it back into soil. Earthworms feed on leaf litter, pulling leaves into the ground for feeding—in a sense, they are micro-tilling the soil in a way that is beneficial to soil structure—and depositing their castings, a biologically rich mixture of mineral particles, organic matter, and living bacteria, underground. This helps speed up the nutrient cycling of that litter, making the nutrients within the plant matter available to the growing plants more quickly. This is just one illustration of how plant matter changes from a living thing to fertilizer for the next generation of plants to come.
What if, as another example, you surface-apply compost to your pastures? How will it get down to the roots of your forage plants without tillage? Nature provides the answer—in natural ecosystems, organic matter is always applied from the top down. Think of leaves falling to the ground in the forest, or grasses being bent, trampled, or blown over in the grasslands. The soil organisms and gravity, little by little, carry organic matter down from the soil surface into the soil profile. Earthworms and other tunneling organisms encourage downward movement of nutrients. Water-soluble nutrients are carried into the soil when it rains. When plant roots encounter compost, they can’t just take a big bite and assimilate it. They need the soil microorganisms to break down organic matter into the most basic building blocks—a process called mineralization. Organic matter has to travel through the digestive systems of many players in the food chain before it can go from tough lignin, for example, to glucose, K+, Ca2+, NH4+, Mg2+, etc. that plant roots can take in and use to fuel their growth. If any of these biotic players are missing from the soil food web, nutrient cycling becomes less efficient. This is why it is critical to focus on the soil biology in order to improve nutrient cycling. We will present the details of the groups of species that make up the soil food web later in this publication.
Organic Matter
There are two ways organic matter can be added to the soil: through the roots as exudates, and through the decomposition of plant and animal residues. The second of these two, the “breaking down” process, is probably the more familiar concept to most people. The traditional belief has been that humus forms when organic matter decomposes into its most basic components. Then, in a not-fully-understood process called “humification,” it forms large, stable molecules in conjunction with the mineral portion of the soil. However, there are some in the soil-science community who now point to recent studies suggesting humus formation in the soil comes primarily from the anabolic process of root exudates (Jones, 2015). It was as recent as 1993 that glomalin was identified by researchers at the United States Department of Agriculture (USDA); glomalin is an organic exudate produced by mycorrhizal fungi that contributes greatly to soil structure and, likely, to the formation of humus (Magdoff and Weil, 2004).
It is important to remember that no matter how humus is added to the soil, it is critical to foster all processes that result in the continual addition of organic matter to the soil. To keep roots exuding sugars as much of the year as possible, we need to maximize photosynthesis, lengthening the growing season as well as making the plant canopy as dense, diverse, and layered as possible. In a factory analogy, this would be keeping the factory operating as much of the day and year as possible. In addition to maximizing root exudation, it is important to continually feed organic material to the beginning of the disassembly line to keep the workers in the factory, i.e., the soil food web, consistently supplied with the material they need to do their work: cycling nutrients.
Types of Organic Matter
Organic matter can be categorized into several types. First, there is the organic matter that is called either “green” or “living.” This is the organic matter that is currently a part of living plants, fungi, and micro- and macroorganisms. It is unavailable for plant uptake, since it is bound up within the living bodies themselves.
The next part of organic matter is called brown or dead. This is organic matter that is often still recognizable, such as leaf litter, wood chips, or animal bodies. This organic matter is at the beginning of the disassembly process. It will feed the soil organisms and move through the soil food web, from secondary to tertiary consumers. As it is broken down, it becomes closer and closer to its most basic parts and pieces, and thus becomes closer and closer to being plant-available.
The next category of organic matter is black or very dead. This is generally considered to be humus, highly resilient to weathering and closely bound up with the mineral portion of the soil, protecting it from further decomposition. A final category is “recalcitrant” organic matter, which is also called biochar or charcoal. This is found in terra preta soils in the Amazon, which are pockets of enriched soils created by pre-Columbian peoples as a way to enhance the fertility of the poor, highly weathered tropical soils there. Terra preta soils are less acidic and more nutrient-rich than the native soils that surround them. Studies suggest that biochar, which has a high surface area, improves the soil’s ability to hold cations and improves soil structure (Lehmann and Joseph, 2009). Biochar in the soil has been seen to persist for thousands of years, conferring benefits to the soil for that long. Because of this, people have once again begun using biochar as a soil amendment to increase soil fertility, pH, and fungal activity (Phillips, 2017). This form of organic matter is unlikely to be found in most soils unless it is intentionally added. For more information about biochar, see the ATTRA publication Biochar and Sustainable Agriculture.
While the scientists continue to do the important work of discovery about the mysteries of humification and organic-matter accumulation, farmers can do the important work of putting to use what they’ve already discovered:
- Maintain actively growing plants as much of the year as possible.
- Avoid erosion at all costs.
- Continually add plant matter to the front of the disassembly line to be moved through the soil food web.
These practices will help improve the soil health of our pastures. They keep the carbon flowing down into the soil food web, keep the soil well structured, and assure that plants are well supplied with the nutrients they need to thrive.
Abiotic Soil Properties
The abiotic, or non-living, portion of the soil includes what most people think of when they think of soil—the mineral portion. Many of the qualities of the mineral portion are considered “inherent” properties, and cannot be altered through management. The nature of the mineral portion of the soil depends on what parent material it originated from and how the soil formed. Soil texture, which is the percent of sand, silt, and clay present in the soil, is something most people are familiar with. You may have heard terms like “sandy loam” or “silty clay loam” to describe various soils—those terms are describing the soil’s texture. Soil texture cannot be changed through management, but it must be considered when making management decisions. A soil with a high percentage of clay, for example, will take longer to dry out after a rainfall event than a soil with a high percentage of sand. Therefore, those areas may need longer rest periods after rain before allowing cattle to graze. Other considerations are how soil texture affects a soil’s ability to retain organic matter. Sandy soils are notorious for being naturally low in organic matter. Therefore, it will take greater effort on the part of the manager to increase the percentage of organic matter in those soils.
Some soils are naturally higher or lower in certain mineral compositions, based on what type of rock they originated from, but with few exceptions, most agricultural soils generally have within them enough nutrients to support productive plant growth (Ingham, 2014). The problems arise when the soil biology has been depleted so that the plants are no longer able to access these nutrients—the nutrients are there, but in unavailable forms. If forages are continually overgrazed, the roots and mycorrhizae will have so little energy coming in from the few leaves left behind that they won’t be able to reach untapped regions of the soil. Plants and fungi won’t be able to exude plentiful sugar and protein complexes into the soil to attract soil microbes, and therefore both their delivery mechanisms and the “brown” organic matter digesters will be less plentiful. In short, the microbial bridge becomes non-functional.
Without soil biology, soil would be a structureless, lifeless accumulation of small mineral particles (think the desert, or Mars). When life enters the picture, soils become enriched with all the exudates, glues, sugars, humus, and living things that make up the soil ecosystem. These are what give soil the ability to form aggregates. These aggregates, in turn, help soil absorb water and exchange air, allowing roots and soil organisms to respire.
Soil pH
Soil pH is the measure of acidity or alkalinity of soil, and is one of the most important soil chemical properties to consider in terms of nutrient cycling. Soil pH greatly influences nutrient availability: many plant nutrients are most available for plant uptake at a slightly acidic soil pH of 5.5 to 6.5, though legumes thrive in 6.5 to 7.0. Low-pH soils (less than 5.5) can cause aluminum toxicity in plants. In high-pH soils, many nutrients (P, Fe, Mn, Bo, for example) become unavailable (Brady and Weil, 1999). Soil tests will estimate your soil’s pH, but keep in mind that this is a dynamic property that can change based on how much rainfall your soil has experienced, time of year, and how the pasture has been managed. The soil-test results are a snapshot in time, and although it is important to know the pH of your soil, make sure you take the conditions in which you sampled into consideration.
Management practices can alter soil pH. Lime and organic matter increase soil pH and decrease soil acidity. Soil organic matter adsorbs positively charged ions, including hydrogen ions (H+) that cause soil acidity. Lime increases soil pH (decreases acidity) by displacing acid-forming hydrogen and aluminum bound to the edges of soil particles and replacing them with calcium or magnesium. Limestone that is finely ground is most effective in altering soil pH, because it has more surface area to bind to soil particles. All commercial limestone has label requirements that specify its capacity to neutralize soil pH and its reactivity, based on the coarseness or fineness of grind.
Lime refers to two types of materials, calcium carbonate and dolomite. Dolomite is a combination of calcium and magnesium carbonate. Calcium carbonate is recommended for soils low in calcium; where grass tetany or magnesium deficiency is an animal-health problem, dolomite limestone should be used. In sandy soils or soils with low to moderate levels of potassium, the calcium or magnesium in lime can displace potassium, reducing its availability. Therefore, other methods of increasing soil pH may be preferable in those soils.
Lime can be an expensive soil amendment. If your soils have a low pH, you can try to increase it to a more favorable level by changing management practices to those that increase soil-organic-matter content. Increasing organic matter can significantly improve soil pH. It may take a few years to see improvement, but it will also likely be a long-term pH change that will keep pH relatively stable over time.
Synthetic Fertilizer and Soil pH
Throughout this publication, we encourage enhancing biological methods to improve soil fertility, and pH is another reason to do so. Application of some synthetic nitrogen fertilizers acidifies soils. Soil microorganisms involved in nitrification rapidly transform urea or ammonia into nitrate. This nitrification process releases hydrogen ions into the soil solution, causing acidification, which decreases nutrient availability, thus slowing the growth of plants and soil organisms. Another fertilizer that acidifies the soil is superphosphate. Superphosphate forms a highly acid (pH 1.5) solution when mixed with water. The impact of this acidification is temporary and only near where the fertilizer was applied, but, in this limited area, the highly acid solution can kill rhizobia and other soil microorganisms.
The Base of the Soil Food Web: Bacteria and Fungi
The food web in the soil is structured like the more familiar food webs in ecosystems above the soil. Although the specifics of who-eats-who are different in different ecosystems, food webs are structured in similar ways in terms of trophic levels. This is often depicted in a pyramid, with plants at the base, herbivores above them, then the predators that feast on the herbivores, and at the top of the pyramid, the apex predators. Underlying all of this are the decomposers: the true base—organisms that are superabundant and expert at breaking down nutrient compounds and holding them in simpler forms. They are the most nutrient-dense organisms on the planet. This base makes nutrient cycling possible; passing nutrients from one player in the food web to the next, with the “extras” being excreted as waste products that often happen to be plant-available.
Bacteria and fungi are the decomposers in the soil food web. They break down and consume complex minerals, carbohydrates, proteins, and fats with a startling array of enzymes. Once inside the cell, these plant nutrients are immobilized, only to be released when these primary food-web members are eaten by predatory microbes. Billions of protozoa and nematodes flit around consuming bacteria and fungi, releasing not only N-P-K, but all plant nutrients (the list of essential plant nutrients keeps growing, so you may as well include all of the minerals on Earth). This unceasing war among organisms in the soil underworld supports plant life. And while it may seem that plants are passive observers of this activity that surrounds them, plants in fact exert their own influence on the soil organisms by altering their exudates in accordance with their specific nutrient needs.
Bacteria
Bacteria and archaea are the most numerous organisms in the soil. Scientists believe that there are hundreds of millions of species, and most of them are not yet discovered. After fungi, they are the second-greatest decomposer in the soil community. Bacteria are immobile unless they can hitch a ride on some other organism or float on a micro-current of water. However, what they lack in mobility, they make up in sheer numbers. In one teaspoon of good soil, there are between 100 million and one billion bacteria (Lowenfels and Lewis, 2010). Remember that picture of the Milky Way? In the soil, the concentration of brightness is the rhizosphere, in large part due to the millions of bacteria clustering around plant roots, feeding on plant exudates. Bacteria can reproduce at phenomenal rates and also never really die from old age—they just go to sleep when conditions are unfavorable and wake up when they improve.
Archaea, similar to but not technically the same as bacteria, are thought to be one of the most prominent converters of ammonium to nitrate (the process is called nitrification) in the soil. Because archaea and bacteria are so similar in function, this publication refers to them all together as bacteria.
Bacteria further influence their surroundings by producing a biofilm, or bacterial slime. This sticky slime prevents desiccation and protects them from being washed far down into the soil profile during a heavy rain or irrigation event. It holds them in the resource-rich rhizosphere. Bacterial slime is also slightly alkaline, raising the pH in the micro-niches of the rhizosphere. This facilitates nutrient cycling. True to the complex interrelationships in soil, the slime that bacteria produce also facilitates the movement of protozoa and nematodes and creates micro-aggregates, fashioning favorable habitats one micron at a time. These micro-aggregates are further grouped and glued together by the glomalin produced by mycorrhizal fungi, resulting in a well-structured soil.
One micron (or micrometer) is incredibly small. It is one-millionth of a meter, or one-thousandth of a millimeter (1mm is slightly less than 1/16 of an inch). A bacterium cell is typically one micron in diameter. A soil fungal hyphal cell can range from one to several microns in diameter. In the soil, biological, physical, and chemical events can easily be conducted within the sphere of one micron. There are nearly 13,000 microns in half an inch, the radius of the rhizosphere around a single root—imagine the geography!
Bacteria exude powerful enzymes through their cell membranes into the biofilm that surrounds the nutrient substrate the bacteria are trying to consume, such as organic matter. Much like different keys to different locks, each enzyme is chemically suited to break down a certain long-chain molecule, be it a carbohydrate, protein, lipid, or mineral complex. The denatured components of these large molecules then move through the cell membrane into the cell by both simple diffusion and active transport. There they remain until a predator protozoa or nematode comes along, eats the bacteria, and releases them as waste products, ready for plant roots to take up, or for fungi to gather and take directly to the roots themselves.
Fungi
Fungi are the giants of soil infrastructure. They decompose the greatest amount of biomass in the soil. They are also more specialized in their structure than bacteria. Unlike bacteria, many fungi are multi-cellular. They are composed of long carbon pipes, called hyphae, which are made of chitin and have cell walls called septa. At the end of the hyphae, fungi have an actively growing apical tip that can penetrate cell walls and inject powerful enzymes to denature the cell contents and ingest them into the hyphae.
Consider the hyphal advantage: fungal hyphae can grow at a rate of 40 micrometers a minute. In contrast, bacteria may spend their entire existence within an area only six microns in diameter. With their hyphae, fungi can transport nutrients to plant roots from above-ground decaying organic matter and also from organic matter deep within the soil. One of the attributes that makes fungi so beneficial to pastures is this spatial range that allows them to gather nutrients from large expanses of the soil ecosystem.
There are basically two kinds of fungi: the decomposers, called saprophytes, and those responsible for nutrient transfer, the arbuscular mycorrhizal fungi (AMF). AMF have a symbiotic relationship with plants through their hyphae, which form a direct pipeline to facilitate nutrient and water transfer between plant roots and the surrounding soil.
This symbiotic relationship increases the access that plant roots have to the soil profile by a hundred times. In pastures with thick swards of both grasses and legumes, AMF are very beneficial, transporting nitrogen, phosphorus, and a host of other plant-available nutrients directly into the roots, and even allowing plants of different species to exchange nutrients. This means that if you have well-established AMF in your pasture soil, your nitrogen-fixing legumes can share that N with the grasses next to them. And grasses, which are good at getting phosphorus (P), can share that P with the legumes. The AMF allow diverse species of plants to support each other.
Additionally, fungi emit oxalic acid, which serves as a cation exchange site. Calcium is often bonded to oxalic acid. If your soils need calcium, increasing the fungal population is beneficial. Mycorrhizal fungi also contribute to soil-aggregate stability by producing glomalin. This protein holds soil micro-aggregates together, builds soil structure, and gives healthy soil all of its beneficial traits: water infiltration, aerobic pores, cation exchange capacity, and, most importantly, a house for microbes.
Cation exchange capacity (CEC) is a measure of how many negative charges on the surfaces of soil particles (either mineral or organic) are present to adsorb cations, i.e., positively charged ions such as Ca2+ or NH4+. The higher the cation exchange capacity, the more soil nutrients can be held. CEC is often given as an estimate on soil tests, listed as the estimated cation exchange capacity, or ECEC, and is based on soil texture, which is an inherent soil property. Fine-textured soils generally have higher CEC than coarse-textured soils. CEC can be increased, however, by increasing the percentage of organic matter in the soil. This will increase the soil’s capacity to hold nutrients within reach of the plant roots.
Moving from a scale of microns to a scale of miles, fungi are the largest organisms on earth. Fungi have been found in the Olympic Rainforest in Washington to measure 20 miles in length. In a fantastic yearly display of growth and predation, this fungus grows to gigantic proportions each winter, only to be almost totally eliminated by predation from protozoa and nematodes during the spring and summer. Think of the amount of plant nutrients this yearly cycle releases into the soil.
Saprophytic fungi (the decomposers) are adept at decomposing “hard” carbon, the tough cellulose and lignin found in mature grasses and woody material, turning them into more simple compounds ready to be further transformed by the rest of the food web into plant-available nutrients. As we shall see, this has advantageous implications when managing our pastures to maximize nutrient cycling.
Fungi:Bacteria Ratios
Scientists have observed that the ratio between fungi and bacteria in the soil (F:B ratio) changes based on land use. In forests, F:B ratios can be anywhere from 5:1 (deciduous forest) up to 1000:1 (coniferous forest). In grasslands, F:B ratios are closer to 1:1. Poorer or more-disturbed soils are typically more bacterially dominated, with ratios of less than 1:1. One reason for this is that fungi are more sensitive to disturbances such as tillage, and their numbers can rapidly decrease when these disturbances occur. The type of plant residue that is available for decomposition can affect the F:B ratio as well, because fungi favor plant residues high in cellulose, and bacteria favor residues with a lower carbon-to-nitrogen ratio (C:N ratio), such as green plant materials or legume residues.
The ratio of F:B can be an indicator of where your soil lies along the line of ecosystem succession. The typical soil test run by many laboratories will not measure the F:B ratio of your soil. However, you can have this measured by the Earthfort laboratory (see Further Resources for contact information), if you are curious about your soil biological makeup and what it says about the condition of your primary soil-nutrient cyclers, i.e., the bacteria, fungi, protozoa, and nematodes.
One key fact must be kept in mind with bacteria and saprophytic fungi. While they are alive, they do not immediately release nitrogen or any other element that they have consumed. It is as if those nutrients are in the bank. So, then what? We have all of these plant nutrients locked inside microbes. How are they transformed into nutrients that are plant available? Through predation.
The Predators: Protozoa and Nematodes
Protozoa and nematodes are the sharks of the soil-microbe world. They are in near-constant movement, with each one consuming 10,000 bacteria a day. In healthy soils, there are 50,000 protozoa per gram of soil, gobbling up 500 million bacteria per gram of soil each and every day. The sheer scale of consumption directly beneath our grass canopy is difficult to grasp, but it is what drives the nutrient movement on which we rely.
Protozoa include flagellates, amoebas, and ciliates. A healthy, functional soil will have many more flagellates and amoebas than ciliates. Ciliates are an indication of anaerobic soils, where metabolism takes place in the absence of oxygen, and that is something that you do not want. Not only are anaerobic soils dominated by pathogenic bacteria, fungi, and nematodes, they do not cycle any plant nutrients, and plants will starve.
Carbon: Nitrogen Ratios in Soil Organisms and Agricultural Materials | |
Organism | C:N Ratio |
Wheat straw | 80:1 |
Rye Cover Crop (Anthesis) | 37:1 |
Mature Alfalfa Hay | 25:1 |
Ideal Microbial Diet | 24:1 |
Hairy Vetch | 11:1 |
Soil Microbes | 8:1 |
Source: USDA NRCS |
The soil food web is driven by organisms’ quest for carbon, the basis of life. Bacteria, fungi, nematodes and protozoa all consume carbon to support the biochemical processes needed for biological function. In the table above, C: N ratios of various organisms are listed per unit of biomass. Organisms must maintain these ratios by excreting excess nutrients. For example, protozoa, which have a C:N ratio of 30:1, have a diet of bacteria (which have a C:N of 5:1) that leads to an excess of N. When they consume 30 units of C by eating bacteria, they consume 6 units of N, which is 5 units too many. They then excrete these 5 units of N. They cannot keep the excess N in their bodies or else they will die. The N is excreted as ammonium (NH4+) ions. Thus, plant-available nutrients are born—or rather, recycled. It is nature’s obligate propensity to balance itself that makes minerals available to plants.
Due to the huge concentration of microbes in the rhizosphere, these now-mobilized ammonium ions are easily absorbed directly by the root or are delivered into the root by mycorrhizae. Some ammonium ions do not get absorbed immediately by plants or fungi. Instead, nitrifying bacteria convert the ammonium into nitrate N, (NO3–). Because nitrate-N has a negative charge, it is more vulnerable to leaching and may leave the system before plants are able to use it. Additionally, other ammonium ions are incorporated into protein complexes. These additional forms of nitrogen can be actively absorbed by roots or brought into roots by mycorrhizal fungi.
The process of consumption and excretion of nutrients is going on every second of every day, supplying minerals to our pasture plants with just-in-time service. Plant nutrients not taken up by the plants are immediately reabsorbed by bacteria and fungi. In nature, nutrients are rarely in such excess that they are just floating around with nothing to consume them—a big difference between nature and inorganic fertilization.
As graziers, then, we need to ask the next question: can healthy soil supply all the needs of our pasture plants to grow several tons of forage? Some estimates indicate that the predation of soil bacteria by protozoa can release up to 35 times the nitrogen need of plants (Ingham, 2014). Since all of this occurs in the rhizosphere, plants are able to access the nutrients they need, and there is enough to feed the abundance of microorganisms that reside there as well. There is no leaching. Yes, we absolutely can grow high-producing pasture solely by maintaining the natural nutrient cycle.
The key here is this can happen in a healthy soil. What makes soil healthy? It has to have an adequate amount of active microorganisms and the organic matter, i.e., carbon, to support them. It also needs enough healthy plants per square foot to supply the exudates needed to feed the bacteria continually.
Can this happen in 2% organic matter soils? They have insufficient carbon, so probably not. Can this occur while fertilizing with large amounts of inorganic N? Definitely not. Inorganic N fertilizers shut off root exudates, and their salt composition kills microbes through desiccation.
Notes on Nitrogen: Legumes and Biological Nitrogen Fixation
If you have healthy soil, nitrogen becomes plant-available through the predation and excretion of plant-available nitrogen by soil organisms, as we have just described (this process is called mineralization). But if the soil you are starting with is poor, and you are looking to increase N levels to help boost productivity, what is the best way to do this? Commercial fertilizer N is damaging to pasture soils, and getting enough N through compost application can be expensive.
There is another, renewable source of N that farmers have utilized for millennia: biological nitrogen fixation. Biological nitrogen fixation is done primarily by bacteria in association with legume plants, but also by certain soil bacteria such as actinobacteria and cyanobacteria. Establishing or maintaining legumes in the pasture while providing good habitat for free-living, nitrogen-fixing bacteria is a cost-effective, long-term way to ensure pastures have sufficient N. Biological nitrogen fixation provides approximately 65% of the nitrogen used by crops and pastures globally and can be maximized by maintaining diverse plants and robust agricultural soils (Jones, 2014).
Legumes—clover, alfalfa, vetch, peas, and many more plant species—add nitrogen to pasture systems though nitrogen fixation, the process of converting atmospheric nitrogen (N2) into plant-available forms. Legumes are able to do this because they form a partnership with a type of bacteria called rhizobia. Rhizobia bacteria colonize legume roots, forming nodules, where they fix nitrogen and feed it to the plant in exchange for energy. The legume plants use this nitrogen as they grow.
Nitrogen can move from legumes into the rest of the pasture system through several different pathways. If the legume is eaten by livestock, they digest and absorb some of the N, but much of it is returned to the soil via manure or urine. If the plant goes uneaten and dies, N can enter the soil as the plant decomposes. Some of the N will enter the soil directly through the roots, as they grow and die back, and as nitrogen-rich exudates (Brady and Weil, 1999). If the pasture soil has a robust mycorrhizal fungi network, legume plants and grasses can be connected at the roots by fungal hyphae, and nitrogen can be shared between them. This is yet another reason to encourage plant diversity in your pasture.
One additional benefit of having legumes in a pasture is that they improve the nutritive value of the pasture, resulting in healthier livestock with increased gain (Hopkins, 2000).
Carbon Runs It All
We don’t have to know how the myriad of plant nutrients (N, P, K, Ca, S, Mg, Na, B, Cl, Mn, Zn, Cu, Mo, etc.) are chemically transferred from one trophic level to the next—it truly is a miracle of biochemistry, the details of which will not need to be considered when thinking about grazing management strategies. As graziers, we just need to remember that it all starts with the fourth-most abundant element in the universe: carbon. Without carbon, life stops. That is why a large pool of soil organic matter, which is 60 to 70% carbon, is so important to have in our soil. Without it, there is not enough biological activity to carry mineral elements on the journey through the soil food web toward becoming plant-available. With carbon, the rest of the nutrients can cycle rapidly, and we have the potential to grow high yields of quality pasture forage without inorganic fertilizers. Carbon is where nutrient cycling begins.
Macroorganisms: Earthworms and Dung Beetles
Much of this publication has been about all the activity in the soil that we can’t see, but there are visible indicators of a healthy pasture-soil ecosystem, and we will discuss two of those now: earthworms and dung beetles. These two creatures play important roles in cycling nutrients, and the more that are present in your pasture, the better.
First, let’s consider earthworms. Earthworms are integral to a healthy pasture ecosystem, improving soil structure and increasing the rate of nutrient cycling. Their importance to agricultural productivity has been known for millennia. Earthworms were called “earth’s intestines,” by Aristotle, and Cleopatra decreed them sacred animals and made it illegal to harm them or remove them from the Nile region (Feller et al., 2015). Charles Darwin wrote an entire book on earthworms late in his life and stated, “Without the work of this humble creature, who knows nothing of the benefits he confers upon mankind, agriculture, as we know it, would be very difficult, if not wholly impossible” (Darwin, 1881).
Why do earthworms receive such praise? There are many ways earthworms improve the soil ecosystem. First, they feed on organic matter and are critical to incorporating organic matter from the soil surface into the soil profile. One study in Australia showed that in a grassland without earthworms, the thatch of plant matter at the surface became more than 1.5 inches thick, but when earthworms were introduced, that thatch layer was reduced as the earthworms incorporated it into the soil (Magdoff and Weil, 2004).
Second, earthworms speed nutrient cycling in the soil. The shredding action of earthworm digestion increases the surface area of the organic matter, making the organic matter more readily available to soil microorganisms that feast on the earthworm feces, or castings. They also increase soil bacteria populations, in a few ways. Like livestock rumens, they host microorganisms in their gut that help them digest, and their casts have high populations of bacteria. Additionally, when earthworms move through the soil, they exude mucus that feeds soil bacteria. The portion of soil affected by earthworm activity is called the drilosphere, much like the area of soil around roots is called the rhizosphere. The drilosphere includes the passages earthworms create when they move through the soil, their casts, and the biologically enhanced soil just surrounding these holes. The drilosphere has more biological activity than the surrounding soil—picture another Milky Way in the galaxy of soil life.
The third significant way earthworms improve the soil is by developing soil structure. Their passages are like superhighways for air and water, as well as for root growth.
There are approximately 2,700 different species of earthworms around the world, but most that exist in North America can be divided into three basic categories based on where they live: epigeic worms live in the litter at the surface and do not burrow into the soil; endogeic worms burrow several inches into the soil; and anecic species are deep burrowers (nightcrawlers being a familiar example). Having a diverse population of earthworms in your soil will improve the efficiency of nutrient cycling and will increase the volume of soil improved by their activities.
Because earthworms are a visible indication of soil health, as opposed to the billions of invisible lifeforms present, they are often used as a way to monitor soil health. Tracking earthworm populations is a good way to see the current state of your soil ecosystem and to chart it over time, by keeping notes of your findings and comparing them from one year to the next. A basic method for monitoring earthworms is to dig a spadeful of soil and break it apart to look for earthworms, tunnels, casts, and other macroorganisms. It is best to do this in spring or fall, when the soil is moist but not saturated. In general, if you only see one or two earthworms, your soil is probably in need of more organic matter. If you see between two and 10 earthworms, you’ve got a medium level of soil health, and if you see more than 10, your soils are probably in very good health (Magdoff and Van Es, 2010).
This is a great example of how improving soil organic matter levels will lead to a positive feedback loop of soil improvement. Good pasture management will increase soil organic matter, and will therefore increase earthworm populations. Having more earthworms will improve your soil resource further, which will lead to even more earthworms, and even richer soils, and even better pasture, on and on.
Dung beetles provide some services to the soil similar to earthworms and should be of particular interest to graziers. Here is a quick summary of their many benefits, drawn from the ATTRA publication Dung Beetle Benefits in the Pasture Ecosystem (Thomas, 2001):
- Increased pasture yields resulting from the incorporation of organic matter into the soil, with an increase in soil friability, aeration, and water-holding capacity
- Reduction of insect-pest populations that breed in animal feces
- Prevention of pasture surface pollution
- Reduction of animal diseases by removing contaminated feces from pasture surfaces
- Return to the soil of nutrients that would otherwise be tied up in fecal deposits and unavailable to pasture grasses
- Increased effective grazing areas of pastures covered by feces
- Reduced nitrogen loss in livestock feces
As their name implies, dung beetles provide these benefits to the pasture system by consuming dung and/or drinking the liquid from the dung (depending on the type of dung beetle), thereby making the cowpats or other livestock feces less suitable for fly larvae or parasites, while making those nutrients available to microbes and plants. They directly deposit the dung to the plant roots by rolling the dung into balls and burying it. This makes it available for the microbes in the soil to start consuming, cycling those nutrients much faster than if they were left on the surface.
Dung beetle populations are susceptible to some dewormers commonly used to control internal parasites in livestock. All administration of Ivermectin, (oral drench, subcutaneous, or bolus) has detrimental effects on dung beetle populations. Using these and other insecticides as sparingly as possible will help keep dung beetle populations up and actively cycling nutrients.
Dung beetles and earthworms represent organisms near the top of the soil food web. Healthy populations of these species and others, such as micro-arthropods and other insects, are important indicators of an invisible, robust microbial community of bacteria, fungi, nematodes, and protozoa that is cycling nutrients in your pastures.
Herbivores’ Role in Cycling Nutrients
While we graziers tend to view our livestock simply as consumers of our pastures, in reality one of their greatest functions is the cycling of plant nutrients in order that our pastures may grow again. Livestock are inherently inefficient in forage conversion. As much as 70 to 80% of the forage ruminant livestock consume is returned to the pasture in the form of urine and feces, which are heavily inoculated with microorganisms.
Herbivores, Grass, and the Reset Button
“Never does Nature separate the animal and vegetable worlds. This is a mistake she cannot endure, and of all the errors which modern agriculture has committed, this abandonment of mixed husbandry has been the most fatal.”
― Albert Howard, The Soil and Health: A Study of Organic Agriculture, 1947
Grasslands represent the most potential of any biological landscape on earth to sequester carbon and other nutrients. Over a century, properly managed grasslands will sequester even more carbon than will rainforests (Salatin, 2017). What allows them to do so is what farmer and writer Joel Salatin aptly calls the “reset button,” that is, the act of an herbivore taking a bite of grass. Before humans and agriculture entered the picture, grasslands coevolved with wild ruminants that were the original reset button. In our managed pastures, grazing livestock provide the chomp that resets the plants. When herbivores defoliate grass through grazing, the grass switches into high gear, releasing a flush of carbon and protein exudates through its roots to feed microbes in exchange for nutrients. Plants need to maintain a balanced ratio of leaves-to-roots, so when forage is grazed by livestock, they slough off roots. When the plants resume their growth during the rest period, root regrowth occurs as well. The nipped plant continues to grow until it is fully recovered, and that is when the grazier allows livestock into that paddock again, and another round of nutrient cycling commences. Over and over again. You can see how productive grasslands can build rich soil over time; all due to the reset ‘chomp’ that herbivores provide.
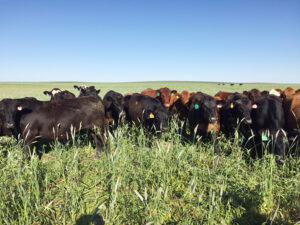
The pasture “reset button.” Herbivores are key to productive nutrient cycling. Photo: Kate Vogel, North 40 Ag
Let’s look more closely at the reset event. As your cows, sheep, or goats take that bite of grass, the saliva itself stimulates certain exudates to be emitted from the plant roots. Talk about millions of years of living together and the adaptation that results! One chomp sets that plant fully into gear. Then where does that bite of grass go? Straight into the livestock’s very own fermentation vat—the rumen. In a controlled environment teeming with microbes, biochemical compounds are denatured and resynthesized to meet the needs of the grazing animal.
But that is only the first part of the cycle. Typically, we think of the process as this: soil grows grass that feeds the livestock. But the cycle continues: livestock feed the soil that grows the forage, as well, and not just with inert nutrients. After the cow or sheep or goat gets its nutrition from the grass (in partnership with the microbes in the rumen), it then returns nutrition and living microbes back to the soil ecosystem it stands on. A wholesome supper of organic and inorganic nutrients is provided to the soil food web. Within the feces is a dose of billions of rumen microbes that, incidentally, are of comparable species to their soil compatriots. Livestock are really just another kind of feed dealer and inoculum supplier. The bacteria, fungi, protozoa, nematodes, dung beetles, and earthworms flock to manure and urine consignments, first immobilizing those minerals and then releasing them as plant-available nutrients. Quite the merry-go-round, and all because of animals with four stomachs and their microbial partners.
Holistic Management and Nutrient Cycling
Whole books have been written on the role of livestock in nutrient cycling. One of the most notable is Allan Savory’s Holistic Management. You can boil down what Allan eloquently says in 500 pages into this basic framework for creating managed grazing: rest, residual, and stocking density. See “Managing the Mature Stand,” in ATTRA’s Managed Grazing Tutorial. This regimen has a direct effect on how mineral nutrients, water, and energy are cycled through the pasture ecosystem and the diversity of the plant community that results.
Adequate pasture rest enables maximum photosynthesis. Maximized photosynthesis allows for increased root exudates, organic matter, microbe populations, nutrient transfer, and subsequent forage growth. Stocking at a higher density, along with sufficient periods of rest, pushes more carbon into the soil through trampling and subsequent decomposition. When you combine rest and intensity, you maximize the liquid carbon pathway and the decomposition of residue, creating diverse pasture ecosystem-building synergy. Furthermore, you will likely see a shift from bacterially dominated pasture soil toward a F:B ratio closer to 1:1, a typical ratio of natural grasslands.
The herbivores are complemented by the actions of their human stewards, who can enhance the system by adding compost. In climes where shed lambing and calving are the norm, the manures and bedding generated provide yet another major way to enhance nutrient cycling in our pastures. In the span of one winter, a tremendous amount of carbon (straw), nitrogen, and other nutrients found in manure can be collected and composted.
Compost and compost teas do wonders to add microbes and, in the case of compost, food for the microbes to our soils. Soils with low organic matter will benefit greatly from repeated surface applications of compost. Compost generated from the waste products of herbivores is perhaps one the most underutilized nutrient-cycling tools on our farms and ranches. If you have to purchase straw, consider it a short-term liability converted into a value-added, long-term asset, i.e., a healthy and fertile soil.
A Functioning Soil and a Profitable Pasture
In healthy pasture soils, nature abounds because of a diversity and complexity that is exceedingly difficult, if not impossible, for us to fathom. However, we can behold plainly the beauty it produces: productive pastures that are abundant with few outside inputs. As we move to more holistic methods, our pastures do not require—in fact, they are damaged by—inputs of inorganic fertilizer and pesticides. The animals that graze the holistically managed pasture are healthier, with less parasitism and less nutritional imbalance, spurring greater gain. The biology in our pastures is a three-ring circus, a moving kaleidoscope of activity, and, as graziers, we hold the powers of the ringmaster, keeping the whole show together.
Managing for Optimal Pasture Nutrient Cycling
How do we get this magical three-ring circus going in our pastures? As graziers, we start off with a huge advantage in that we can limit or completely eliminate tillage. Long-lived perennial pasture provides a stable base to promulgate a more balanced and productive soil ecology. Of course, every farm has its own set of circumstances, but in many instances we do not need to reseed our pastures annually or even every several years, or can do so using no-till technology. Eliminating tillage serves to build aggregation and enhance soil-microbe proliferation, especially fungi, as both saprophytic and mycorrhizal fungi are significantly damaged by tillage. Grazing our paddocks for short durations, leaving behind sufficient residual, and following with adequate rest periods can eliminate the need to reseed. Many progressive graziers have proven this to be unequivocally possible.
Against this perennial backdrop, we can employ three practices to promote nutrient cycling: living roots, high stock density grazing, and compost.
Living Roots
First, we can always ensure that there is a living root and adequate plant cover present in our pastures. In the northern regions of the United States, this means leaving behind sufficient residual to cover any bare soil, even in the winter months. In the south, the critical period switches to the summer: here we can utilize warm-season perennials to produce forages that provide soil cover and living roots, as well as overseed cool-season forage species in the same pasture to ensure growth for as much of the year as possible. In both climes, managing residual correctly during the active grazing season and moving livestock from paddocks with at least six to eight inches of grass not grazed will keep the plants active and keep the roots exuding carbohydrates.
For a discussion of adequate pasture rest and how to know when you your grass has achieved it, see the ATTRA Managed Grazing tutorial.
Another management strategy is turning out the livestock one week later in the spring, giving the grass a little more time to put on some growth, and thereby increasing the grazing wedge (See the “Managing the Mature Stand” lesson in ATTRA’s Managed Grazing tutorial). Tall grass amplifies the amount of plant sugars and proteins that are exuded to soil microbes, greatly increasing their populations and the plant nutrients that they, in turn, funnel back to the plants. The result is greater productivity. And remember, the liquid carbon pathway is critical for building humus in our soils. Using an enhanced liquid carbon pathway, i.e., tall grass, to sequester carbon in the form of humus dramatically increases soil organic matter, bringing about the factors that enhance nutrient cycling.
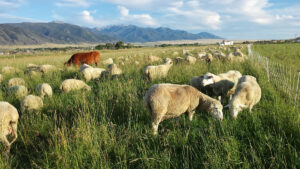
Tall-grass grazing, as opposed to “vegetative grazing” is simply allowing grass paddocks to recover fully before turning livestock back in to regraze. Some graziers extend the rest period even a little more to reduce parasite larval viability and to enhance soil health by providing increased amounts of food for fungi (cellulose) and plant exudates. Photo: Dave Scott, NCAT
Tall-grass grazing promotes root growth and density. This is because the plants are never grazed before they are fully recovered. Additionally, there are two other nutrient-cycling benefits of grazing tall grass. First, mature, taller grass “snaps” and stays bent close to the ground when trampled. Soil organisms can then get hold of it. Vegetative grass is so limber that it tends to pop back up when stepped on, greatly decreasing microbial access. The second benefit is that mature, trampled grass encourages the growth of soil fungi, because the fungi are best equipped to break down the complex carbohydrates such as cellulose and lignin that compose more of mature grass.
At the time of writing (2017), publication author Dave Scott’s farm, Montana Highland Lamb, located outside of Butte, Montana, had pastures with a soil organic matter level of 5.5%. In 1981 it was 1.3%. Levels have risen from 5.0% to 5.5% in the last four years of grazing tall grasses and leaving behind 50% as trampled residual. Additionally, the fungi:bacteria ratio has increased from 0.30 to 0.69 during the same time span. While not yet to the desired 1:1 ratio, the trend is in the right direction, with fungal numbers doubling in that time period. Thus, one way to increase soil fungi is to graze and trample tall grass. No inputs are required; just a change in the way you graze. You may lose a little at first in daily gain, but in subsequent years, as the ecosystem adjusts, that loss will be recovered. This has been the case at Montana Highland Lamb.
Animal Health Benefits
Cattle will feast on the top of the grass plants, where a higher percentage of the energy is located. Scissor-billed sheep are ideally equipped to select tall grass leaves and combine them with the right amount of cellulose-laden stems to balance their fiber needs. The result is lower blood-urea nitrogen levels and less of other metabolic disorders such as infertility, mastitis, laminitis, and liver dysfunction. Are your cows negatively affected by washy grass? How about your sheep with fly strike? Do you have any idea on how much you spend on fly tags in a lifetime? Efficient nutrient cycling promotes increased animal health and a whole spectrum of decreased inputs, be they soil, plant, or animal. All of these add up to more-profitable animals grazing grass of higher return.
High Stock Density Grazing
The second tool to increase nutrient cycling is to graze with high stock densities. This is nature’s way to distribute ingested nutrients evenly across the landscape. We all have seen the results of a large paddock stocked at a low density: much of the urine and manure is found in a small part of the field, i.e., adjacent to the water source, or underneath the shade trees. Almost invariably, those nutrient-laden sections are also overgrazed, creating an even greater imbalance in pasture nutrient cycling. The combination of over-fertilization and over-grazing can damage these areas, can be a potential source of nutrient runoff, and deprives other areas of needed nutrients. Additionally, there is little trampling of plants because low stock densities result in plants being eaten before they can be trampled. Proper grazing with high stock densities solves these problems.
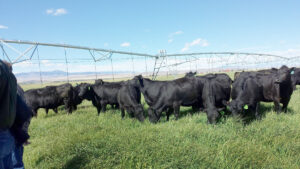
High stock density grazing is not difficult to implement. At La Cense Ranch in Dillon, Montana, 200 of these heifers (160,000 pounds of live weight per acre) are moved every day. Moving time? The one-wire fence is moved and the heifers are into the next paddock in 12 minutes, flat. Photo: Dave Scott, NCAT
High stock densities also shift the fungi: bacteria ratio toward 1:1, which, as we have discussed, is the ratio found in natural grasslands, suggesting that this is a balanced way to cycle nutrients for long-term productivity (Masters, 2017). We will profit by mimicking nature: cashing in on the closed loop of an intact nutrient cycle that will require no outside inputs of fertility. Simultaneously, we will build soil organic matter faster than scientists believed possible only a few years ago.
What is an acceptable stocking density to make all of these positive changes in nutrient cycling? How much live weight per acre? Those that are improving their soil the fastest are employing stocking densities of 500,000 pounds of live weight per acre or more. One-half million pounds of live weight per acre is equivalent to 830 yearling calves per acre, weighing 600 pounds apiece. This grazing group will consume 14,940 pounds of dry matter in a 24-hour period. If you have 4,000 pounds of dry matter in your one-acre paddock, and you plan to leave 50% residual, 2,000 pounds of dry matter will be grazed in three hours. Hello, Batt Latches!
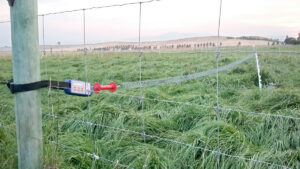
A Batt Latch is a solar-powered automatic gate opener developed in New Zealand. It retails for about $400. You can set the time when the latch will open. Livestock quickly become accustomed to the opener and move into the new paddock when it releases the spring gate.
Here’s an example that takes it down a notch. Say we are willing to move daily, and we have 4,000 pounds of dry matter (grass) per acre. We are irrigated and we are also aiming for 40-day rest periods. We can use the ATTRA Grazier’s Calculator to easily determine that we can put 110 six-weight yearlings on one acre. That works out to 66,000 pounds of live weight per acre.
While this certainly is not one-half million pounds of live weight per acre, it is a practical real-life situation. It will also lead to improved nutrient cycling over time, as has been seen at Montana Highland Lamb, where this kind of real-life rate of high stock density grazing has been implemented.
If you are groaning at the prospect of moving cattle every day, it really is not that bad. If you have to be gone for an extended period, all you have to do is set a few Batt Latches or just give your livestock a larger paddock while you are gone. It won’t be the end of the world. When you get back, resume your daily moves. What you do for your pastures by moving every day will far outweigh an occasional large paddock.
One of the big benefits of moving every day with higher stocking densities and long rest periods is the accompanying influx of diverse plant species that will become established in your pastures. Be patient and this will happen! Nature’s succession always tends to diversity and diversity begets stability. A diverse sward of forages above ground guarantees a diverse microbial population below. Why? Because different species of plants emit a variety of exudates which nurture a whole spectrum of bacteria, fungi, nematodes, and protozoa (Stika, 2016). Monocultures limit the complexity and brightness of that rhizospheric Milky Way. The large masses of mycorrhizal fungi that are present in a diverse pasture facilitate nutrient transfer and water absorption. This can be readily seen in a drought, when resilient, diverse pastures stay green and productive longer and rebound more quickly than neighboring monoculture pastures. Diversity is also a benefit for you in periods of less stress, producing more tons of dry matter for your livestock. A diverse pasture that has the support of a diverse microbial base also contains more forage nutrient density. This not only leads to greater gains, but also higher quality meat. We need diverse pastures.
Compost
Adding good compost or compost tea to your pastures in place of inorganic fertilizers is the third major practice you can utilize to improve nutrient cycling. Compost and teas derived from it inoculate the soil, augmenting the initial microbial populations. As many graziers have experienced, compost applications can result in immediate production responses that are sustained for three or more years. How many applications are needed and how long they last is a function of how well your soil is functioning biologically. At Montana Highland Lamb, Dave Scott tries to cover each acre once every three years. On areas of the farm that have poorer soil, he has observed that compost applications increase grass production.
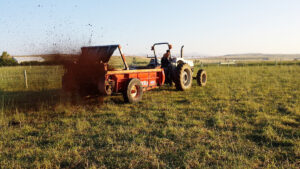
Compost supplies nutrients and microbial inoculants all in one application. Additionally, it can be used to alter the fungi:bacteria ratio. Photo: Dave Scott, NCAT
In a study conducted on California grasslands, a single application of compost to grasslands increased carbon storage 25 to 70%, and that is not including the carbon that was in the compost that was applied (White, 2014). And while carbon dioxide respiration increased, as well, increases in plant production offset the increase in respiration, meaning carbon was being added to the soil for the three years of the study after the initial single application of the compost, with no sign of stopping once the study was completed. The increase in carbon storage can likely be attributed to an improvement in plant productivity: plants were able to pump more carbon into the soil than they were before compost was applied.
Compost inoculants are also a great tool to increase soil fungi. Creating a fungal-dominated compost or tea on a large scale is not as easy as producing a bacterially dominated compost, but it is possible. For more information on composting, see the ATTRA publication, Tipsheet: Compost and the video, Composting! You Can Do It. Both can be downloaded from the ATTRA website.
What About Hay?
Many hay meadows never see the hooves of the livestock they feed. Therein lies the hidden cost of hay. The hay is removed and fed to livestock elsewhere. All the nutrients that were in the hay are exported from that field and need to be replaced somehow. Frequently, this is done using commercial fertilizer, but over time the soils become depleted of organic matter under this style of management. One solution is to incorporate hay fields into a rotational grazing system, so that these fields can be treated to the stimulating livestock massage of “chomp,” controlled trampling, and manure fertilization. The only problem is that if you feed hay for more than a few months out of the year, you probably will have too many hay acres to “recondition.” And, to further complicate things, if you produce hay for only two months of feeding, you probably can’t justify the machinery expense. Thus, many graziers have sold the swather, figured out ways to graze longer into the winter—or in some climes, through the winter (see the lesson on Stockpiled Grass in the ATTRA Managed Grazing Tutorial)—and have bought the hay that they need. Not only is this a boon to ranch economics, it will actually serve to import nutrients onto your farm.
How you feed these imported nutrients to your livestock will determine their overall effect. Hay can be a feed, an organic fertilizer, and, in some cases, a source of seed. Manure from feeding hay in a feedlot can be composted and returned to hay fields. Or, to make the whole process simpler, consider bale grazing. Livestock take their share and soil microbes relish the residue. Rolling out bales to cover large sections of a field and grazing with high stock densities will feed cows, feed microbes the trampled hay and, if the hay has mature seeds, will plant grass, as well. Perhaps the least efficient manner to feed hay is to feed it on the hay field and make the cows eat it all between feedings. The only thing left for the microbes is the manure. It is better than nothing, yes, but think of all that carbon you could have added by feeding more and trampling it in. From a nutrient-cycling standpoint, feeding hay just to cows and not to microbes does not repay the full hidden mortgage of producing stored feed.
The Whole Show
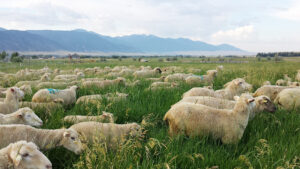
The winning team: tall grass, high stock density, and good compost. Photo: Dave Scott, NCAT
Now that we have added tall-grass grazing, increased stocking density, and good compost to our grazing operation, what can we cut out? Once your pasture soil is functioning efficiently, you will not have to fuss with inorganic fertilizer anymore. Soil microbes have taken over that job for no pay.
You won’t have to use herbicides to control weeds because your livestock will do it for you. You will be using fewer fly tags because pasture biology will be tending to that chore. You may even be taking fewer trips to the feed store for mineral because your forages will be more nutrient-dense. Those graziers who have chosen this pathway also don’t have to deworm their livestock nearly as often, if at all. All for the same amount of quality grass. Does this sound like a good trade?
Ratcheting Down Commercial Nitrogen While Retaining Grass Production
In her immensely informative article, Nitrogen: The Double-Edged Sword, Dr. Christine Jones (2014) outlines a path to reducing commercial nitrogen in pastures over a five-year period. She recommends a 20% reduction in the first year, followed by two years of additional 30% reduction, culminating with two years of minimal applications of 5 kg N/hectare (4.5 pounds per acre). At Montana Highland Lamb, the Scott family has roughly followed her advice for more than four years, and so far it has proved successful. There has been no detectable drop in grass production, and this year (2017) they have not applied any N fertilizer. The soil organic matter level is 5.5%.
How much soil organic matter (SOM) is prerequisite to successfully ratcheting down your N fertilization? Currently, there is little research exploring this question, but experience suggests that 4% SOM would be an absolute minimum. If you don’t have that level of SOM, you have two options. First, you can graze what you have with high stock densities. This is what Dr. Allen Williams has done on his ranch in Mississippi. You can read about his success in regenerating poor soil in his article in the The Grassfed Exchange (2015). Secondly, you can plant and graze multi-species cover crops for three or four years. Graze them with the goal of improving soil health. Specifically, graze one-third and trample two-thirds with high stock density grazing. Perhaps the best strategy is to combine the two methods, generating enough cash flow with your existing livestock numbers while your soil improves. Perhaps you can only afford to put 10% of your ground in covers each year. That’s fine; you will get across your farm eventually. Increasing pasture soil’s health and function is a long-term investment, yielding big dividends over the years.
Under the Big Top
Living roots, grazing with high stock densities, and good compost are the management keys to improving nutrient cycling in our pastures. With them, we are collaborating with the natural soil-building power of nature rather than trying to supplant nature with expensive chemical and technological solutions. Nature always has the last word. Working with such a powerful force ensures ultimate success. Seeing the resulting natural abundance truly is the greatest show on earth.
Nutrient Cycling’s Effect on the Water Cycle
As you might expect, efficient water cycling goes hand-in-hand with effective nutrient cycling. The increased soil aggregation, a byproduct of abundant soil life, will increase water infiltration rates and the soil’s water-holding capacity. Therefore, it will be less likely to lose nutrients either through runoff or through leaching into groundwater. Another positive-feedback loop will ensue, as an enhanced water cycle will benefit the microbial populations that mineralize plant nutrients. The water cycle is so integral with the nutrient cycle that one simple way to tell if you are cycling nutrients well is to perform a simple water-infiltration test. Which brings us to monitoring.
Plot Your Progress: Monitor
Monitoring the outcomes of the grazing, trampling, and composting processes you employ is essential for two reasons.
First, it inspires hope to see the land respond positively when you work more harmoniously with nature. Comparing this year’s spadeful of soil with one from two years ago is excellent motivation when you note a deeper brown color, better crumbly structure, more earthworms, and a denser package of roots. These are signs that you are on the right track. They give us all hope.
Second, monitoring is the gateway to adaptation. Monitoring illuminates the unexpected, which will almost certainly happen. Inserting a change in one place on the biological chain often elicits a reaction somewhere else in the system, which manifests itself perhaps where you would not expect it. Do not despair; learn to adapt by looking at the entire ecosystem and keeping an eye toward the future.
Case Study
In 2014, the Scott family at Montana Highland Lamb thought they had managed to curb the Barber Pole Worm infection in their flock by increasing the rest period from 22 to 32 days, moving daily, and by leaving behind six to eight inches of residual. They were so confident, they decided to move on to the next critical issue affecting their profitability: fertilizer inputs. They increased their rest period to 42 days and grazed taller grass, trampling in significantly more forage to feed the microbes. What they did not expect was a parasite resurgence. However, that is precisely what happened. Parasite infection in 2015 included more than 200 cases in 480 lambs and ewes, some of which were multiple. This is up from 20 in 2014. This was discouraging to say the least. Did they bail out of this new management strategy? Or, instead, try to analyze what they could potentially employ to address the surprise negative outcome? The Scotts decided to continue on their path and address the issue of animal stress. They implemented a fenceline weaning program to reduce lamb stress and potentially also reduce the parasite infection rate. It turned out to be a positive move. In 2017, parasite infections decreased to 54. Monitoring through FAMACHA scoring gave them the ability to quantify the results of a shift in management. It also allowed them to address the issue in a holistic manner, based on their observations and the data they had diligently gathered.
Without monitoring, moving toward enhanced nutrient cycling in your pastures will be a game of chance rather than a game of strategy. Sustained progress without monitoring may be elusive. For more on simple pasture monitoring (including the water-infiltration test), see the Monitoring section in the free ATTRA Managed Grazing tutorial. Monitoring only takes an hour or two a year, and the payoff is certainly worth it.
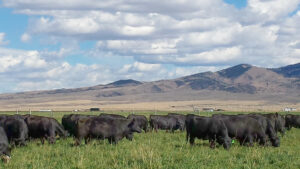
Black Angus cattle on intensively managed pasture in Montana. Superior gains with low inputs = profitability. Photo: Dave Scott, NCAT
Conclusion
Carbon and water: these two elements drive soil nutrient cycling. With sufficient carbon, all the players in the pasture ecosystem flourish. You have maximum photosynthesis that allows the liquid carbon pathway to hum like a dynamo, root exudates proliferate, fungal and bacterial partnerships form, organic matter in the soil increases, pH stabilizes, and microbes mineralize nutrients continually. This is nature’s template.
As you consider your strategy regarding nutrient cycling in your pastures, you face a fork in the road. You really only have two choices. One, stay with the status quo of fertilize, fertilize, and commit to endless years of more commercial inputs and their associated drain on your pocketbook and the environment. Or you can take the other path and gradually transition to an approach of working more with nature to utilize all the complex biological resources available to supply plants with the nutrients they need. Living roots, long rest periods, high stock density and the application of quality compost are the grazier’s tools to intensify the liquid carbon pathway and to enable efficient nutrient cycling. Once your pasture soils begin to function well, your pasture yield will be equal to or greater than conventionally fertilized pastures. You will need less and less commercial fertilizer and pesticide. Your pastures will infiltrate and use all of the moisture they receive. They will sequester huge amounts of carbon. Profit will result. It won’t happen overnight, but it will happen. Nature’s plan has a mountain of inertia behind it. Use it.
References
Brady, N. and R. Weil. 1999. The Nature and Properties of Soils, Twelfth Edition. Prentiss Hall, Inc., Upper Saddle River, NJ.
Darwin, Charles. 1881. The Formation of Vegetable Mould Through the Action of Worms, With Observations on their Habits. London, England.
Feller, C., E. Blanchart, and G.G. Brown. 2015. Popularity of earthworms before and after Darwin. The International Union of Soil Sciences.
Hopkins, Alan. 2000. Grass: Its Production & Utilization, Third Edition. Blackwell Science, Ltd., Malden, MA.
Howard, Sir Albert. 1947. The Soil and Health, A Study of Organic Agriculture. University Press of Kentucky, Lexington, KY.
Ingham, Elaine. 2014. The Soil Food Web. Proceedings from workshop. Montana Chapter of the Soil and Water Conservation Society, Billings, MT.
Jones, Christine. 2014. Nitrogen: The Double-Edged Sword.
Jones, Christine. 2015. Save Our Soils. Acres USA. Vol. 45, No. 3.
Lehmann, J., and S. Joseph (eds.). 2009. Biochar: Environmental Management. Earthscan, Sterling, VA.
Lowenfels, Jeff, and Wayne Lewis. 2010. Teaming with Microbes. Timber Press, Inc., Portland, OR.
Magdoff, Fred, and Harold van Es. 2010. Building Soils for Better Crops. SARE.
Magdoff, Fred and Ray Weil. 2004. Soil Organic Matter in Sustainable Agriculture. CRC Press, Boca Raton, FL.
Masters, Nicole. 2017. Soil Crawl: Getting Down and Dirty. Proceedings from workshop. Northern Plains Resource Council. Big Timber, MT.
Phillips, Michael. 2017. Mycorrhizal Planet: How Symbiotic Fungi Work with Roots to Support Plant Health and Build Soil Fertility. Chelsea Green Publishing, White River Junction, VT.
Salatin, Joel. 2017. Why animals are important. Stockman Grass Farmer. July. p. 28.
Stika, Jon. 2016. A Soil Owner’s Manual: How to Restore and Maintain Soil Health. Jon Stika, North Dakota.
Thomas, Michelle. 2001. Dung Beetle Benefits in the Pasture Ecosystem. ATTRA Publication CT155.
White, Courtney. 2014. Grass, Soil, Hope. Chelsea Green Publishing, White River Junction, VT.
Williams, Allen. 2015. Recent data supports soil health benefits of adaptive high stock density grazing. The Grassfed Exchange.
Further Resources
ATTRA Case Studies: Increasing Soil Health through Cover Crop Cocktails
No-Till Case Study, Brown’s Ranch: Improving Soil Health Improves the Bottom Line
Explains Gabe Brown’s approach to soil management, focusing on soil health and the use of no-till farming, diverse cover crops, and intensive rotational cattle grazing on his North Dakota farm.
No-Till Case Study, Miller Farm: Restoring Grazing Land with Cover Crops
Ken Miller of Mandan, North Dakota, discusses how converting marginal cropland back to grazing land can be accomplished by planting several years of a diverse cover crop mixture to help break up the old plow layer and increase nutrient cycling.
No-Till Case Study, Richter Farm: Cover Crop Cocktails in a Forage-Based System
Marlyn and Patrick Richter, who farm in North Dakota, grew a multispecies cover crop cocktail after an early forage harvest to add needed residue, organic matter, and available soil nutrients for the subsequent cash crop. Their forage-based cropping system had routinely removed most plant biomass from the land, resulting in inadequate plant residue for healthy soil-biology function and soil protection.
ATTRA Publications and Projects
Dung Beetle Benefits in a Pasture Ecosystem
Dung beetles play a small but remarkable role in the pasture ecosystem. They feed on manure, use it to provide housing and food for their young, and improve nutrient cycling, soil structure, and forage growth in the meantime. This publication describes dung beetles and their benefits to pastures and offers management information.
Grazing Planning Manual and Workbook
This manual provides all the resources you need to write your own grazing plan, monitor its efficacy, and adjust your management throughout the grazing season.
Integrating Livestock and Crops: Improving Soil, Solving Problems, Increasing Income
Incorporating livestock into a crop farm (grain, vegetable, or orchard) can benefit soil organic matter and fertility, diversify the product base, provide new sources of income and farm resilience, and help with weed and pest control. This publication outlines some of the benefits and challenges of integrating livestock into a farm and offers tips and resources to ease the transition.
Managed Grazing Tutorial
This tutorial features sessions taught by National Center for Appropriate Technology specialists who are also livestock producers. They share years of experience managing their own pastures to inspire you to start wherever you are and build or refine your own managed grazing systems. Detailed presentations and real-world examples will get you on the road to managed grazing.
Soil Health Tutorial
This tutorial introduces key soil health principles and discusses simple on-farm soil health assessments that farmers and ranchers can perform themselves.
Nutrient Cycling Resources
Amazing Carbon. By Christine Jones, PhD
Articles on soil health and management by a soil ecologist who assists farmers and ranchers enhance biodiversity, increase biological activity, sequester carbon, activate soil nutrient cycles, restore water balance, and improve productivity. The articles titled “Liquid Carbon Pathway” and “Nitrogen: The double-edged sword” are of particular relevance.
Creating Healthy Soils with Holistic Management. By Holistic Management International
Videos and articles about improving soil health and function through planned grazing.
Farming in Nature’s Image. 2015. By Gabe Brown.
A presentation at the Connecting Soils and Profits: No-till, Cover Crops, Soil Health, and Grazing Conference held in Danville, Pennsylvania, March 18, 2015.
Soil Biology Primer. 2000. By A.J. Tugel, A.M. Lewandowski, and D. Happe-vonArb (eds.). Soil and Water Conservation Society, Ankeny, IA.
This is the online version of the book, offered at no charge by NRCS. It’s a beginning treatise on the
food web.
Soil Carbon Cowboys. 2013. By Peter Byck.
A video with ranchers Allen Williams, Gabe Brown, and Neil Dennis discussing how they regenerated their soils while improving animal health and profitability.
Nutrient Cycling in Pastures
By Dave Scott and Nina Prater, NCAT Sustainable Agriculture Specialists
Published March 2018
©NCAT
IP136
Slot 49
This publication is produced by the National Center for Appropriate Technology through the ATTRA Sustainable Agriculture program, under a cooperative agreement with USDA Rural Development. ATTRA.NCAT.ORG.