Cover Crop Options for Hot and Humid Areas
By Justin Duncan, NCAT Agriculture Specialist
Photo: Subtropical Organic Agriculture Research (SOAR)
Abstract
Cover crops can be better adapted to some regions better than others. This publication discusses the characteristics of cover crops that are better suited for areas with hot, humid summers, like the southern portions of Texas and Florida and along the Gulf Coast, the Caribbean, Hawaii, and points beyond with similar climatic conditions. It includes a table that will allow you to make the best decision for your situation about which cover crops may suit your individual needs. It also includes a general inoculant guide for legume crops.
Contents
Introduction
Sub-Tropical Cover Crop Options
References
Further Resources
Appendix 1: Inoculant Groups
Introduction
One challenge of organic crop production in hot humid places is proper cover-crop selection and management. Which covers are most appropriate? Which can take the heat? Too many times the available answers aren’t a proper fit for hotter, more humid areas. This publication will explore some options for USDA Plant Hardiness Zones 8 and above. An interactive Plant Hardiness Zone Map can be found here.
Cover cropping is especially important in hot places because soil organic matter volatilizes at faster rates in hotter areas than in cooler areas. Cooler areas have more organic matter but are more sensitive to losing that organic matter in higher temperatures. For instance, in a cool area one can expect a 10% loss of organic matter, but in a warmer region only 3% loss, if the mean temperature rises 1° Celsius. What this suggests is a stratification of resiliency of soil organic matter. Consider, for instance, woody materials: they take longer to break down than leaves. So, as organic matter is deposited in the soil, the more resilient materials are the last to break down, while the other materials—in descending order of toughness—decompose at accelerating rates in direct relation to the rising ambient soil temperature. So, in hotter soils there is less organic matter, but they aren’t as sensitive to temperature increases as cooler soils. This is very important because as soils in cooler regions lose organic matter, they also lose the ability to retain water, which contributes to warmer ambient temperatures (Kirschbaum, 1995).
Because decomposition in hot, humid soils is so fast, it takes about twice as much input of soil organic matter to replace organic matter lost through volatilization and harvest of crops. Thus, a producer in a hot, humid area is fighting three battles with soil organic matter, and all are uphill. First is the heat, which saps the organic matter from the soil. Next is the sheer amount of material it takes to restore fertility to the soil each season or growing cycle, and third is maintaining enough soil moisture to retain the limited soil organic matter that is there. In no-till systems, about half as much soil organic matter is lost through decomposition as in conventional tillage (Vieira et al., 2009).
Organic matter in soil also regulates decomposition of the available nitrogen and carbon and conserves them in a relative manner: i.e., the more soil organic matter, the more carbon and nitrogen the soil can hold, which increases soil microbial activities. Another effect of higher soil organic matter is that it disperses minerals that can have negative effects, such as iron, by binding to them in the soil-organic-matter matrix (Bayer et al., 2000). This and other metals can be toxic in higher concentrations in the soil and inhibit root growth. In acidic soils with toxic levels of manganese, organic molecules such as cysteine and tannic acids, which are readily found in plant materials, can be helpful in reducing the amounts to less harmful levels (Hue et al., 1999). Another highly toxic metal found in acidic soils, aluminum, has also been found to be relatively ‘disarmed’ by organic acids found in the soil. These bind enough aluminum to influence root growth significantly (Hue et al., 1985).
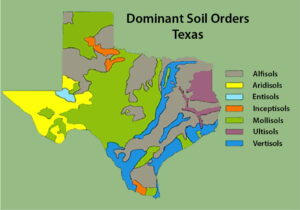
Figure 1. Bands of soil orders in the eastern side of the state are representative of soil deposition during changes in sea level. The higher-elevation western portion of the state does not exhibit the banding effect. Map used with permission of USDA NRCS.
Although soil organic matter (SOM) is very important to soil health (Gosper and Murray, 2003), it is harder to maintain in hotter areas. The options to build SOM are varied. Producers can add compost or manure, use no-till, or incorporate cover crops into the rotation and later into the soil. Cover cropping generally makes use of non-cash crops to cover the soil in order to prevent erosion and weed build-up and to generate and regulate soil organic material. Most literature in the United States about cover crops is concerned with more temperate regions, so information about covers for hotter areas, like the Rio Grande Valley in Southern Texas, is sparse. This environment is more sub-tropical than temperate, more like southern Florida or southern California. Areas such as these are seldom bothered by frost, so cover crops that do well in other areas, like vetch and Austrian peas, may not do as well (Jeuffroy et al., 1990). Instead, more-tropical cover crops can be useful in these areas because they offer longer growing seasons and provide more heat units than are available in more temperate regions.
One consideration in choosing a cover crop is how it performs in your soil. You must keep in mind that even if a cover crop generally performs well in your region, your specific soil type may be very different than the cover’s ideal conditions. This is common in places like Texas, where there are bands of soil types deposited at different ecological times.
Figure 1 illustrates that in the southern tip of the state, the Rio Grande Valley has four main soil orders, with the Vertisols separated into two major regions, one along the coast and the other along the Rio Grande River and its tributaries in South Texas. These soil differences explain why some vegetation types are found in one place and not others.
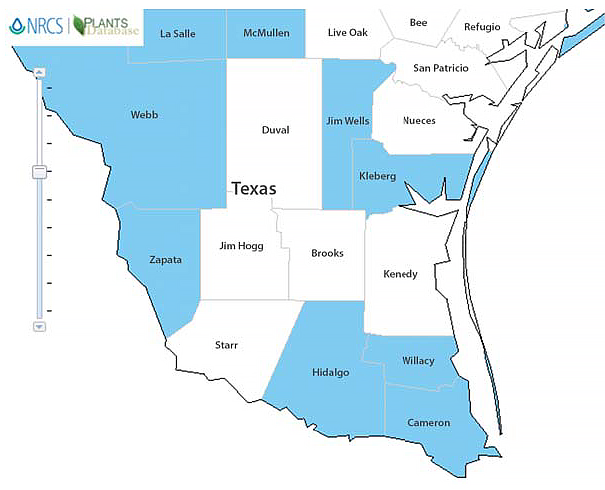
Figure 2. Known distribution of Medicago polymorpha (bur clover) in South Texas. Graphic: courtesy of USDA NRCS.
When we compare Figures 1 and 2, we can see that although the weather patterns are similar across the area, M. polymorpha is not found in all counties. This could be attributed to some of the soil types not being conducive to the growth of that particular species.
Water is also a very important consideration. Rainfall patterns and fluctuations in those patterns can determine whether or not your cover crop is successful. There have been cases when expected rains did not fall after fields were seeded, resulting in patchy germination of the cover crop. This allowed weeds to establish in the transitioning field, defeating the purpose of the cover crop. One way to prevent this situation is to mix seed. Some covers require more moisture not only for germination, but also in the initial growth phase. A mixture of seeds could be used to overcome climatic constraints or moisture limitations during initial planting. Once the cover crops have taken the moisture they need from the soil, then they conserve soil moisture through shading, acting as a living mulch (Salako and Tian, 2003).
Another consideration is what the end use of the cover crop will be. Many times, cover crops are tilled under to capitalize upon the release of their nutrients back into the soil and to add organic matter that will improve tilth and soil health. Some producers opt to leave the terminated cover crop in place so they can use the residue as mulch for the following crop. Residue resilience plays a big role in the usefulness of a particular crop as a cover, because some covers are much more substantial than others in this regard. Austrian winter peas, when grown in the humid southern United States, break down very quickly and are relatively soft. Seed can easily be drilled into this cover crop’s residue. Conversely, a crop like Iron-and-Clay Peas leaves a more robust residue and needs to be mowed with a brush hog before the field can be used. Additionally, seasons matter with cover crops. Austrian winter peas are cold-season annuals in the humid South. By May they have usually completed their life cycle, leaving a field ready to be planted. Iron-and-Clay peas, on the other hand, are a warm-season annual that will abundantly produce vegetative material until frost kills them. Mowing prior to frost may not be enough to stop this vining powerhouse from resprouting. It is critical to know the crop, the soil, and the cover crop very intimately, because many factors will influence these on the farm. Keep records to know what worked best and when. For more information, consult the ATTRA publication Scheduling Vegetable Plantings for Continuous Harvest.
Because this publication is focusing on hotter areas, the cover crops discussed here are strictly for places with at least a 9- to 10-month growing season. For these cover crops to be effective, they must be able to perform their best. The dangers of planting them in areas with inadequate time to grow is that they don’t persist and won’t complete their life cycle, nor will they fix the amount of nitrogen that is expected, because their lives were cut short by the weather (Muir, 2012).
Many cover crops are legumes, which means they require a rhizobium inoculant before planting. While the young roots are developing, the Rhizobium ‘infects’ roots by first cooperating with the plant to form infection threads. These tubules allow the rhizobia to move from the outside of the plant to the root cortex where the bacterium ‘sets up shop’ to produce nitrogen-fixing nodules. These rhizobia are host-specific and usually require an inoculant to ensure their presence in the soil. In many cases, rhizobia will form nodules on a legume outside of its host range, but nodules will not function properly and instead of fixing nitrogen will act as a sort of disease on the plant (Gage, 2004). Functioning nodules are almost always some shade of pink when broken open, while non-functioning nodules are grey, tan, or brown when opened. It is very important to purchase the appropriate rhizobia to accompany legume cover seed, in order to encourage the right symbiosis to ensure crop productivity. In clover, this can mean a seven-fold increase in dry matter over the uninoculated clover. Other legumes show similar trends in production when accompanied by the appropriate rhizobia (Bailey, 1915).
Permanent Cover Crops
In some situations, it is beneficial to have a permanent planting of a cover crop, also called a perennial ground cover. This is especially true of established orchards. Ground covers contribute to both the health of the soil and the health of the trees. There is a problem, however. Legumes create problems for themselves in nature due to their nitrogen-fixing habit. Legumes are usually pioneering species that do well on disturbed ground. Once they enrich the soil with nitrates, grassy competitors take advantage and usurp control of the area. For a legume to be used as a permanent cover, it has to be able to out-compete the weedy grasses. One example of a cover crop that can do this is the perennial peanut, which is discussed in more detail later in this publication.
Rhizobium nodulation can be negatively affected by many different factors, which in turn causes a drag on yield. This yield drag is compounded because the factors that affect nodulation usually also affect the plant in negative ways. For example, low soil temperature reduces nodulation because the genes that determine nodule initiation are inactivated by decreasing temperatures. Soil salinity can also affect rates of nodulation. Salinity effects can differ vastly from species to species of legume and also type of rhizobium. For example, Acacia’s and Prosopis’ Rhizobia, interestingly enough, are sensitive to chemicals in the soil, and the presence of those chemicals will reduce their efficiency and efficacy in inducing nodule formation (Fox et al., 2007).
Another benefit of cover crops is that they can provide habitat for beneficial insects, which can use the cover crops as a rallying area to visit adjacent fields to attack their prey (Wang, 2012).
Cover crops can also help suppress weeds within a crop rotation. Many cover crop species exhibit allelopathy, which is the suppression of one plant’s growth by another plant. This can be useful in weed suppression, as with the use of ryegrass or corn gluten meal to suppress weeds, but it could be detrimental if the wrong cover crop is used, such as certain legumes with cotton. Cotton seedling emergence was depressed to 60% with incorporation of varying amounts of hairy vetch and crimson clover residue (White et al., 1989) and there was also a 30% reduction in cotton yield (Khanh et al., 2006).
Pests
Often, the question of cover crops harboring pests arises. There have been cases where populations of stinkbugs or other pests have built up in cover crops and subsequently moved to other fields to damage cash crops (Bugg, 1991). Other literature, like the ATTRA publication Companion Planting & Botanical Pesticides: Concepts & Resources, shows that timing and crop selection can be extremely effective at attracting pest predators and also at repelling pests in some cases.
Although they play a role in suppressing weeds, legume cover crops can conversely increase yield for subsequent or companion crops. Legumes accumulating nitrogen for the subsequent crop is the point of growing cover crops, or at least one of the main benefits. Yield and yield components should be affected in a positive manner, and the degree to which a particular cover crop affects yield is a consideration. It’s known that legumes fix nitrogen and that other crops benefit from that nitrogen in varying ways. However, it is important to remember that each legume fixes a different amount of nitrogen and releases it at a different rate. These rates depend not only on the species of cover crop but also the stage of development of the crops to be used. One study found that, after one year, Mucuna deeringiana, also known as velvet bean, yielded almost 4,500 pounds per acre of dry matter but, after three years, due to lack of persistence and other factors, they only recovered 117 pounds per acre. On the other hand, Aeschynomene histrix, sometimes called porcupine jointvetch, yielded about 850 pounds per acre the first year, and after three years it had amassed an incredible 4,000 pounds per acre of dry matter, which represents a three-fold increase over the second-ranked cover in this study. The aforementioned study planted fallow fields with various cover crop seeds and then measured their growth using a quadrat. After the third year, they plowed under whatever was growing in the plot and grew corn. They found differences in not only the yield of the corn but also the number of kernels on the cobs (Okpara et al., 2005).
Some plants, though tremendous biomass generators, are not appropriate as cover crops due to difficulty in controlling their spread. Aeschynomene, like kudzu, is excluded from this publication only because of its noxious weed status. A list of noxious weeds in the United States can be found here.
Sub-Tropical Cover Crop Options
Peanut relatives (Arachis spp.)
Perennial peanuts (A. glabrata) are native to South America. This plant is different from its more well-known cousin A. hypogaea in that it’s most often propagated from its rhizomes, rather than by seed. It can cross with other closely related species like A. paraguariensis and A. kretschmeri, giving rise to sterile triploids that only form short rhizomes. Its creeping nature makes perennial peanut suitable as a forage crop and understory ground cover for tropical fruit and nut groves. Without competition it can spread six feet per year, but expect less than a sixth of that with grass competition. Thus, stand establishment is very important when using perennial peanut. Stand establishment is initiated by planting sprigs, much like planting Bermuda grass, and the same equipment can be used (Rouse et al., 2001).
A. glabrata is a multi-use legume. It fixes about 150 pounds of nitrogen per acre per year. It is palatable to livestock and has been shown to be beneficial in weight gain and/or milk production of various species. In addition to providing nitrates to fruit and nut trees and providing protein for livestock, it can also be cut and baled for hay and sold. In some cases, perennial peanuts are used for ornamental purposes due to their showy yellow flowers (Miavitz and Rouse, 2002).
Perennial peanuts prefer well-drained, sandy soils with a pH range from 4.5 to 8 and do not tolerate waterlogging. Generally, A. glabrata needs from 1,000 to 2,000mm (39 to 78 inches) of rainfall a year but can survive on 750mm (29 inches). One variety, Latitude 34, can survive on 450mm (18 inches) a year (Muir et al., 2010).
There is another perennial peanut that is used in Hawaii, A. pintoi, which seems to be the more widely used species outside of the Continental United States. Most of the Australian literature is centered on the Amarillo variety, which is used in orchards there (Hensley et al., 1997). The two species are quite similar for the most part, but they react differently in different situations, like drought and cold. A. pintoi (Seeded Perennial Peanut) produces more seed than A. glabrata (Rhizomal Perennial Peanut), but neither of them produce very much in comparison to A. hypogaea (Gosper and Murray, 2003).
Pigeon pea (Cajanus cajan)
Pigeon pea is an upright trifoliate herb that, if allowed to, can become a small tree in some varieties. It is a weak perennial that is most often used as an annual but can live three to five years with the most productive seed bearing occurring in the first two years. There are several wild relatives of pigeon pea on the Indian Sub-Continent, supporting the idea of it being domesticated in that region ~3,500 years ago. Pigeon peas are a well-known food crop eaten throughout the tropical world and are being researched for their antimalarial properties. They have been traditionally used for that purpose in Ghana (Merel et al., 2004; Duker-Eshun et al., 2004).
Pigeon peas are drought tolerant and highly adaptable. They even perform well in nutrient-depleted soils. This crop has been known to produce a harvest even after others have wilted and withered away. Pigeon peas account for about 5% of total world legume production (Odeny, 2007).
As a cover crop, they have been shown to increase corn yields by almost 33% in Togolese production systems, which average around 90° high temperatures throughout the year (Sogbedji et al., 2006). Pigeon peas incorporate phosphorous from the soil very efficiently from orthophosphates and can even pull phosphorous from soils with aluminum. Its upright habit lends itself to being used as windbreaks in adjacent plots. For instance, in a 1-acre field, the center third of the field can mowed and incorporated into the soil, while leaving the edges intact. This will allow the outside to act as a windbreak or a sort of nurse crop for the center. Later, the sides can be mowed, incorporated and then replanted with either a cash crop or more cover crops (Valenzuela, 2011).
Pigeon peas are good nitrogen fixers that supply quite a bit of that nitrate to the subsequent crops through their residue. Most legumes fix nitrogen, but some impart it to other crops better than others. Tree legumes like Leucaena, Calliandra, and Acacia can, in some circumstances, only convert less than 5% of their nitrates into mineralized forms that other plants can use. Pigeon peas, on the other hand, make about 20% of their total nitrogen available to the next crop. These numbers seem to be environmentally dependent, however, because in other circumstances, Leucaena contributed 12% to 28% of its nitrates to subsequent crops (Palm, 1995).
Partridge pea; Roundleaf Cassia (Cassia/Chamaecrista spp.)
As a cover, Cassia is effective at reclaiming surface-mined areas due to its excellent pioneering potential. Mined areas are low in fertility, organic matter, macronutrients and micronutrients, and especially soil flora and fauna and microorganisms.
Round-leaf cassia is native to the Americas. It’s distributed from Mexico and the Caribbean in the north to Argentina in the south. Most Cassia are small trees, but this type only grows about a meter tall. There are a couple species of Cassia that lend themselves to use as cover crops. The first is C. rotundifolia, which has rounder leaves and can be more prostrate. The other is C. fasciculata or partridge pea, which is upright. The latter is native to Texas, the southeastern United States and Mexico; it can be seen blooming in pastures until frost. It’s relatively untouched by disease and suffers from few pests.
The root was once traditionally used in Native American medicine as a stimulant and to increase stamina (Hamel and Chiltoskey, 1975).
Partridge pea is adaptable to areas where there is adequate drainage, as well as moisture. In other words, they appreciate sandy soils. They also do well on the depleted red-clay soils of southern China. These soils are low in macronutrients, micronutrients, and trace elements, as well as high in aluminum, but nonetheless round-leaf Cassia is productive (Hacker et al., 1997). These red-clay soils are somewhat similar to those found throughout the humid southern United States.
Cassia should not be grazed by cattle while it is in later stages of growth, i.e., after flowering, due to toxicity issues. It is not recommended for horses.
As a cover, Cassia is effective at reclaiming surface-mined areas due to its excellent pioneering potential. Mined areas are low in fertility, organic matter, macronutrients and micronutrients, and especially soil flora and fauna and microorganisms. Cassia can flourish in these conditions and initiate the reclamation process (Gillman, 2014). It fixes nitrogen in good amounts, performing almost as well as an application of 100 pounds per acre N at fertilizing corn planted in its incorporated residue (Tanimu et al., 2007).
Centrosema (Centrosema spp.)
Centrosema is an herbaceous, long-vining perennial with delicate stems and lovely lavender papilionaceous flowers that are the source of the common name: butterfly pea. It’s a twining vine rather than a tendrilled one, meaning the entire stem twirls around the object it’s using for support as it grows. The fruit is a slender, flattened legume that shatters when completely dry, thus broadcasting its seeds. It has both tap roots and fibrous roots that penetrate deeply into the soil, allowing it to stabilize slopes or banks and scavenge minerals from the subsoil (Heuzé and Tran, 2016).
Little breeding work has been done on Centrosema, and landraces from different regions exhibit a varying range of adaptability, so there is potential to develop region- or climate-specific cultivars of Centrosema.
Centrosema pubescens has been studied for medicinal properties. It’s been used among the Ibibio people of Nigeria for burns, and recent research has shown it to have potential use as a wound-healing agent due to its strong antimicrobial and antifungal effects (Ekpo et al., 2011).
Several species are used as cover and fodder crops, like C. virginianum, which is distributed from Argentina to the Southern United States. C. molle (C. pubescens) and C. brasilianum are very similar to C. virginianum in appearance and properties. Centro can handle moisture and waterlogging better than many leguminous cover crops, making it suitable for river bottoms and flood plains.
The yield of Centrosema wildly varies wildly from region to region. In Brazil, it can yield 40 tons of fresh biomass per acre per year (FAO Ecocrop, 2007) or three tons of dry matter, but in Australia’s Queensland, it yielded 13 tons dry matter per acre. This again alludes to the lack of breeding efforts made on this crop and points to its potential for adaptation (FAO, no date b). One Nigerian study showed that Centrosema (brasilianum and pascuorum) was only a mediocre performer out of the 13 cover crops they field tested (Tian et al., 2000). Rainfall was adequate, so there may have been some other conditions present that limited production because their Centrosema plots only fixed 11% of the 135 pounds per acre of nitrogen this crop has fixed in other locations (Tian et al., 2000; FAO, no date b). Later, Tanimu and his group (2007) found that Centrosema incorporated into the soil resulted amongst the highest–of several different cover crops–amounts of nutrition benefits for the subsequently grown corn crop. Centrosema is inoculated with cowpea-group inoculants.
As there are several species of Centrosema available, one should be cognizant of their specific needs. Take, for example, C. brasilianum and C. pascuorum. When in the earliest growth stages, C. brasilianum has a high water requirement, while C. pascuorum has a lower requirement. However, by the mature stage, the water needs of the two species have levelled out (Salako and Tian, 2003).
Like many other legumes, Centrosema benefits from additional phosphorous application in deficient situations. A 60-pound per acre application yielded about 300 more pounds of seed per acre than the unamended plot. Phosphorous application also improved seed weight and doubled dry-matter production and nearly doubled crude protein content of the unfertilized control (Omokanye, 2001).
Butterfly pea (Clitoria ternatea)
Clitoria is very closely related to Centrosema: both are sub-tribe Clitorinae, and indeed their flowers look very similar, as well as their twining, delicately stemmed growth habit. Centrosema is trifoliate but Clitoria is pinnate, having five to seven delicate leaflets. The seed pods of Clitoria are much larger than its cousin but are also relatively flat and narrow. It’s a perennial vine that is intolerant of freezing weather.
Although Centrosema is native to the Americas, Clitoria originated in Africa. There are several synonyms for Clitoria ternatea, such as albiflora, bracteata, mearnsii, tanganicensis, and zanzibarensis, but the literature has mostly settled on the first term. Like Centrosema, there has been little in the way of cultivar development, but luckily it is already naturally widely adapted (Staples, 1992).
As far as secondary uses, Clitoria has been used in Ayurvedic traditional medicine for many years (Mukherjee et al., 2008). In southeastern Asia it is used (flowers) as a natural food coloring, especially for rice. This practice increases the phytochemical content of the rice and enriches the diet with antioxidants (Yusof, 2015). The flowers can also be batter-fried, as in tempura. Another use for the flowers is making blue-colored drinks that change color with the addition of lime juice. It’s a stately ornamental vine that can be used as a screen. Aside from being a good cover crop, it’s also a very beneficial fodder crop for goats and is an excellent pioneer species for disturbed land.
Due to Clitoria’s highly ornamental nature, seed is readily available but may not be specifically adapted for cover crop usage or may not be available in quantities necessary for cover crop needs. In this case, propagation will be important. Clitoria is self-pollinated, like many legumes, and readily sets prodigious amounts of viable seed from one plant.
Clitoria roots are tolerant of short-term flooding but cannot survive with ‘wet feet’ for too long. They appreciate summer rainfall at a rate of about 18 inches, and a mean annual rainfall of about 54 inches. (Conway et al., 2001).
Depending upon environment, Clitoria can yield up to 15 tons per acre dry matter each year if it is managed properly and there are ideal conditions. In drier places, expect less than six tons per acre total production. Over the course of a season, about 700 pounds of seed can be produced on an acre. Due to the indeterminate nature of the crop, flowers and pods can be in many different stages of development at any given time, so harvest will not be uniform (Reid and Sinclair, 1980).
Clitoria benefits from Rhizobium inoculation by the cowpea group of inoculants. It can also, to a limited degree, be colonized by soybean inoculants (Oblisami, 1974).
In addition to supplemental phosphorous, Clitoria also responds well to additional zinc, but it responds much better to manganese and boron (Dayal et al., 2015).
Rattlebox, Rattlepod, Sunn Hemp (Crotolaria juncea)
Over the past few years we have seen a rise in popularity in the use of Sunn hemp. It’s a versatile and useful warm season cover crop.
On-Farm Benefits of Sunn Hemp in Subtropical Organic Farms
Work done in a thesis by Savannah Rugg
Sunn Hemp (Crotalaria juncea) was chosen as a cover crop for my thesis research in multifunctionality of cover crops in south Texas because of its ability to withstand high temperatures and humid climates. The crop was grown in two different farms with different soil types and sub climates. The first farm, Terra Preta farm in Edinburg, Texas, had great germination rate in sandy loam soil. However, with high rabbit pressures the crop was grazed and did not produce a good stand. If rabbits or other herbivores are pests on your farm, then you may consider going with a less palatable legume as a cover crop.
Figure 3.
Number of mycorrhizal spores per 10 grams of soil under each cover crop. Our results indicate that the mycorrhizal spore density was influenced by the cover crop identity. Highest number of spores was found under Sunn hemp followed by Sudan grass and lablab (Sotiet al., 2016).
Number of mycorrhizal spores per 10 grams of soil under each cover crop. Our results indicate that the mycorrhizal spore density was influenced by the cover crop identity. Highest number of spores was found under Sunn hemp followed by Sudan grass and lablab (Soti et al., 2016).
The second plot, Yahweh Farm in Harlingen, Texas, has a clay loam soil and experiences more rainfall than the farm in Edinburg. The plant date was delayed because of heavy rainfall in May and June, so the cover crop was not seeded until July 2, 2015. Sunn Hemp did quite well on this farm and produced 4540 pounds per acre of dry biomass. In terms of weed suppression, there was a cover crop to weed ratio of 4.58 pounds: 1 pound (dry biomass per acre), so the plot was not weed free but the cover crop did outcompete the weed competition. This could be due to the late planting date because a previous trial planted earlier in the year had a much denser stand and less weed presence in field. Sunn hemp did very well at promoting mychorrizae spores in the soil. Compared to the control which had 50 spores/10 grams of soil Sunn Hemp had 187 spores/10 grams of soil.
Sunn hemp resulted in highest nitrate concentration in the soil. However, contrary to our expectation, Lablab caused a decline in the soil nitrate. A possible explanation for this outcome could be the high density of weeds in the lablab plots relative to the other treatments. Similarly, Sunn hemp also out performed other treatments in the conservation of phosphorous in the soil signaling its potential as a warm season cover crop to improve soil health in subtropical agroecosystems.
Table 1. Change in the soil nutrient status after each cover crop (in ppm).
Numbers are calculated by subtracting the pre-cover crop soil nutrient concentration from the post cover crop nutrient concentration (Soti et al., 2016).
Pearl Millet Lablab Sunn Hemp Sudan Grass Control Boron 0.2 0.55 0.9 0.45 0.2 Calcium 1852 1406.5 1427 500 3360 Copper 0.6 0.2 0.4 -0.1 -2.3 Iron 0.1 0.1 0.15 0.15 0 Magnesium 24 28 35.5 13.5 27 Manganese -1 -0.5 0.65 -2.45 -6.7 Nitrate 2 -3 7.5 0.5 2 OM% 0.45 0.87 1.46 1.08 -0.48 pH 0.1 0.05 0 0 0.1 Phosphorus 9.9 19.2 19.3 15.35 6.8 Potassium 142 205.5 205.5 138.5 143 Sulfur 0.4 -14.25 -18.65 -12.8 -32.9 Zinc 0.6 0.5 0.6 0.25 -5.3 Overall, Sunn hemp performed the best to enhance soil biology and chemistry in the summer planting season in South Texas. If a farmer is trying to enhance mychorrizae density, soil organic matter, and nitrates in the soil profile, then Sunn hemp would be a great option for a cover crop. However, if weed suppression is the main service a farmer is looking for in a cover crop, he may want to try Sudan grass or ensure an earlier planting date to provide more canopy cover. Sunn hemp has potential of being a great summer cover crop in the hot and humid climates of South Texas.
Tick-trefoil, tick clover, hitch hikers or beggar lice (Desmodium spp.)
This segmented-pod legume uses hooked hairs to spread its enclosed seeds and is commonly known as ‘tick-clover.’ Like clover, it is a nitrogen-fixing legume, but its growth habit more resembles a less-aggressive version of kudzu. Unlike kudzu, Desmodium is not a noxious weed; in fact, none of the cover crops in this publication are considered noxious weeds by the USDA (USDA-NRCS, 2016).
Desmodium is from South America but has now been naturalized in many humid and highland tropics throughout the world. There are several forms of note, including D. uncinatum (silver midrib leaves) and D. intortum (solid green-leaved). D. uncinatum is more tolerant of flooding, high water tables, and lower pH than the green-leaved form. Neither of these is suitable for clay soils, as they can’t stand to be waterlogged. Conversely they don’t like it to be too dry, either. During dry periods the vines will go dormant, but the plant itself will persist for a time. It’s apparent that well drained soil and adequate rainfall or irrigation is important for Desmodium. It needs non-saline soils pH 5 and above (Hacker, 1992).
Tick-clover is widely introduced; the genus can be found all over the United States, but these two more-tropical forms are not so common. They have some interesting properties that make them desirable. First, as a forage crop, Desmodium intortum contains condensed tannins. Condensed tannins in excess can cause fodder to become unpalatable to livestock, but in the right amounts they can help control Haemonchus contortus in ruminant animals like goats and sheep (Debela et al., 2012). Secondly, Pickett et al. (2014) documented using Desmodium in push-pull farming systems in Sub-Saharan Africa, also known as stimulo-deterrent systems, to reduce damage to corn by lepidopteran stem borers. Then, Midega et al. (2014) showed that it was economically viable to do so, not just on the basis of the corn yield but also the potential harvests from the intercropped Desmodium or other pulses.
Lablab (Lablab purpureus)
This vine is native to Africa and has been used there and throughout Asia for many years. It is a monotypic genus, meaning that there is only one species within the genera: Lablab purpureus, formerly known as Dolichos lablab. While there is only one species, there are several sub species, landraces, and cultivars due to local adaptation. The vines can either be annuals or short-lived perennials. The leaves can be eaten raw or cooked, but the seeds must be cooked to destroy cyanogenic glycosides that can cause vomiting, shortness of breath, debilitation and convulsions. Aside from food, Hyacinth beans are an effective wildlife attractant (deer) and ornamental crop.
As a cover crop, it is quite effective at smothering weeds and fixing nitrogen. Due to its initial slow growth, weeds should be controlled during its establishment. Once it starts actively growing, it is an aggressive competitor and will crowd out and shade newly emerging weeds (Sheahan, 2012). Once cut and dried, Lablab biomass contains around 50 pounds of nitrates per acre.
Some of the climbing types can grow to be 25 feet long, but unsupported in field conditions, they usually attain 40 inches or so in height. Although it’s a cover crop and food source for both humans and animals, it can also be very ornamental. Its flowers are quite showy, and then they are followed by purple seed pods. The seeds are either white or black depending on the variety, and some of the wild types have mottled seeds. The seeds have a peculiar feature: an elongated white hilum. The showy flowers attract pollinators, but the vine is subject to the same complement of insects that attack beans.
Lablab prefers acidic soils of a range from pH 4.5 up to about 7. It doesn’t tolerate flooding very well and can be quite drought tolerant after establishment. It requires a minimum of about 30 inches of rainfall per year (Valenzuela and Smith, 2002). In addition to Rhizobium, Lablab also inoculates successfully with the Vigna group of the Bradyrhizobium. Bradyrhizobium has been shown to enhance yield components of hyacinth beans, such as shoot dry weight and overall yield, without significantly influencing pod size, which means the increased yield was from a higher number of pods produced per plant (Ibrahim et al., 2011).
Hyacinth bean also relieves soil compaction more quickly than grasses. Lablab roots increase interconnected pore space in compacted soils three times faster than grasses like sorghum. Lablab also reduced soil ped size, although sorghum and wheat did not. This research suggests that since Lablab increased porosity and reduced ped size in compacted soil, it would also increase the infiltration rate and reduce runoff, which would also serve to hasten the compaction repair process (Pillai and McGarry, 1999).
Lablab benefits from root associations with vesicular-arbuscular mycorrhiza, especially Glomus mosseae. In field studies, G. mosseae increased dry matter, rhizobial nodulation, and phosphorous uptake. The additional nodulation led to an enhanced uptake of nitrates (Mahdi and Atabani, 1992).
Lablab requires greater amounts of heat units than crops like clover or winter peas. It should be grown in areas suitable for its production, or it will suffer yield depression. A North Carolina study showed that it wasn’t an ideal location for Lablab production. First, they couldn’t get good nodulation, which contributed to poor performance; this stresses the importance of inoculation. Next, the weedy control outperformed the Lablab, and indeed the weeds within the Lablab plot attained more than half of the biomass of the Lablab itself, thus stressing the necessity of early season weeding. Finally, North Carolina may just not get enough heat or UV intensity for the Lablab to thrive. Mucuna was also included in this study, and performed as poorly as Lablab (Creamer and Baldwin, 2000).
Table 2. Some agronomic characteristics of selected cover crops suitable for hot and humid areas.
Species | Zone | Soil pH | Soil Type | Seeding Rate lb/acre | Nitrogen Fixed lb/acre | DM tons/ acre | Erosion Reduction | Weed Suppression | Provides Hay? | Provides Secondary Product? | Grazing? | Soil Compaction | Seed Size | Salinity | Beneficials | Mycorrhiza | Expected Germination Rate % | Termination Time Days | Innoculant | Water Use Stage |
ARACHIS glabrata | 7-11 | 4.5-8.0 | sandy loam | transplant | 100 | 2.85 | high | low | yes | yes, ornamental | yes | releives | 0.1 cm | MT | pollinators | positive | transplant | N/A | cowpea group | establishment:high |
CAJANUS cajan | 9 | 5.0-7.0 | any | 5 | 90 | 2.2 | high | low | yes | yes, food | light | relieves | 0.6cm | MT | parasitoids | positive | 91 | 14-21 | cowpea group | mature: medium |
CASSIA spp | 3-11 | 5.0-7.5 | any | 5 | 100 | 1.87 | high | high | no | yes, medicinal | no | no evidence | 0.3cm | S | pollinators | positive | 48 | 25 | cowpea group | mature: medium |
CENTROSEMA molle/pubescens | 7-9 | 4.9-5.5 | sandy loam | 6 | 250 | 1.64 | medium | high | yes | yes, medicinal | yes | relieves | 0.5cm | S | pollinators | positive | 60 | 10 | cowpea group | intermediate:medium |
CLITORIA ternatea | 7-10 | 6.6-7.5 | any | 12 | 281 | 3.07 | low | low | yes | yes, medicinal, ornamental | yes | relieves | 0.6cm | MS | pollinators | positive | 80 | 14-21 | soy or cowpea | mature: medium |
CROTALARIA juncea | 9b-11b | 5.0-7.5 | sand-loam | 50 | 278 | 2.5 | medium | high | no | yes, fiber, reduces nematodes | no | relieves | 0.6cm | S | habitat | positive | 85 | 4-10 | cowpea group | early: high |
DESMODIUM uncinatum | 13 | 5.5-7.0 | any | 12 | 98 | 2.08 | high | high | yes | yes, medicinal | light | relieves | 0.3cm | S | pollinators | positive | 65 | 4-10 | cowpea group | mature: medium |
LABLAB purpureus | 8-11 | 4.5-6.5 | any | 40 | 220 | 8.9 | high | high | yes | yes, food, ornamental | yes | relieves | 1cm | MS | pollinators | positive | 75 | 7-14 | bradyrhizobium | early: high |
MUCUNA pruriens | 9b-11 | 5.5-6.5 | sandy | 30 | 150 | 4.9 | high | high | yes | yes, medicinal | yes | relieves | 1.5cm | MS | nematodes | positive | 70 | 3-14 | cowpea group | early: high |
PHASEOLUS coccineus | 7-11 | 6.6-7.5 | any | 150 | 125 | 6.99 | high | low | no | yes, food, ornamental | yes | relieves | 2cm | MS | pollinators | positive | 94 | 7 | Bean group | intermediate: medium |
STYLOSANTHES guayanensis | 8-11 | 4.0-8.3 | sandy loam | 3 | 100 | 15.61 | medium | high | yes | no | yes | relieves | 0.2cm | S | repels termites | positive | 90 | 2-5 | cowpea group | intermediate: medium |
VIGNA radiata | 7-11 | 6.2-7.2 | sandy loam | 15 | 80 | 1.65 | high | low | yes | yes, food | yes | relieves | 0.3mm | S | parasitoids | positive | 95 | 5-7 | cowpea group | early: high |
Salinity: MT = moderately tolerant; MS = Moderately Susceptible; S=Susceptible
Disclaimer: this information is based on what research was available at the time. Every field and farm is different so results will vary and will need to be tailored to your unique situation.
Seed weights vary by supplier.
Velvet bean, cowage, cowitch, lacuna bean, Lyon bean (Mucuna spp.)
Mucuna is very versatile and can grow in soils ranging from pH 5 to 8, as long as they are well-drained. These large vines require about 38 to 42 inches of rain each year. Mucuna is capable of fixing up to 150 pounds of nitrogen per acre.
Mucuna is a subtropical legume that produces prodigious amounts of foliage and is most likely native to Southern Asia. There, it is widely consumed after thorough preparation. It is also used in medicinal preparations there. It has been found to contain L-Dopa, which is used for Parkinson’s disease therapy. It has also been called an aphrodisiac, used for scorpion stings, and utilized to treat arthritis. It’s also been used as an anti-diabetic, anti-helminthic, and antibiotic, and it contains anti-oxidant, anti-viral, anti-carcinogenic, and anti-inflammatory compounds. Although they have medicinal properties, velvet beans are more widely known to cause severe itching (Lampariello et al., 2012). The pods and some parts of the flowers are covered in stinging hairs that are used in itching powder. In most parts of the world, velvet bean is not invasive, but in South Florida the plant can be a bit aggressive. However, it is not considered noxious. It is essential that the crop be managed appropriately to prevent weediness.
Mucuna is perennial but treated as an annual in the southern United States. The plants are trifoliate and climbing, somewhat resembling kudzu but with much larger seed pods and seeds.
Mucuna is very versatile and can grow in soils ranging from pH 5 to 8, as long as they are well-drained. These large vines require about 38 to 42 inches of rain each year. Mucuna is capable of fixing up to 150 pounds of nitrogen per acre.
M. pruriens comes in two sub-species and four varieties. The variety of consequence is M. pruriens var. utilis, which does not cause intense itching. Velvet beans were once widely used in the southern United States, but were supplanted by soybeans.
In one experiment in Togo, continuously planting corn was shown to reduce available soil nitrate by almost 60%. By incorporating velvet bean into the corn rotation, there was a net gain of 39% over the control. Not only did the velvet bean replace what the corn crop removed, it also added more nitrate than was used, thus precluding the need for additional nitrate inputs (Sogbedji et al., 2006). M. pruriens was also found to increase corn yields more than urea-based fertilizer in some years. Researchers allowed the velvet bean to grow for 20 weeks, then slashed the vines up to use as mulch for the subsequent maize crop. Their work isolated the nitrogen recovered from the subsequent corn crop. It showed that even though more nitrogen was recovered from the urea-treated plot, the Mucuna-mulched plot yielded more, indicating that the increased yield could not be attributed solely to the nitrate content but must have come from other factors that the mulch imparted to the soil (Tian et al., 2000). This reinforces the notion that organic or regenerative agriculture is a synergistic system rather than just a collection of its individual components. Further bolstering this concept is a study undertaken in Benin that showed corn intercropped with velvet bean had more biological activity in the soil than monocultured corn both with and without NPK fertilization. This added soil-microfauna activity led to a reduction in the numbers of obligate phytophagous nematodes like Meloidogyne and Nacobbus, which are pests of many crop species (Blanchart et al., 2006; Caamal-Maldonado et al., 2001).
M. bracteata is useful in rubber plantations in India and Malaysia. It could possibly be adapted to use in citrus groves in the Rio Grande Valley or Florida and to papaya fields.
Scarlet runner bean, or multiflora bean (Phaseolus coccineus)
Scarlet runners are a bit of an anomaly with regard to the rest of the cover crops in this publication. The others are tried-and-true cover crops according to research, but scarlet runners have the potential to be cover crops according to indigenous use.
Scarlet runner beans are closely related to common beans, Phaseolus vulgaris. They are similar in some aspects but differ mainly in two areas. The first is size: scarlet runner beans are HUGE! The vegetative growth is larger, more vigorous, and robust, and so are the seeds and flowers. The other difference is that the scarlet runners have a perennial starchy root. Some indigenous groups eat these roots, as well as the more commonly consumed beans and young pods (Cairns, 2015). However, some report that the tuber is poisonous and should not be eaten (Ashworth, 2002). It’s likely that there are landraces that have been selected for edible tubers, or that the indigenous people employ various techniques to remove the toxins. They use the same inoculant as common beans, unlike most of the others in this publication, which use cowpea inoculant.
Phaseolus species developed in the Americas and were integral in the lives of many native peoples. Scarlet runners’ life cycle exactly matches that of corn; this was done by design. Their growth habit is suitable and their harvest time is right to be intercropped with corn. This is done throughout parts of Central and South America at altitudes above 1,500 feet. Scarlet runners need a bit more support than common beans, so when scarlet runners are used with corn, the planting density of the beans is reduced to a ratio of 1 bean to 10 corn instead of 1 to 1 as with common beans (Cairns, 2015). Not all tall-growing plants may be used as companions with scarlet runner beans, as Hamburdă et al. (2014) found that there was a degree of yield lag (-70% from mean) when planted with Jerusalem artichokes, but when planted with sunflowers, scarlet runners performed 20% higher than the mean in Romania. In another experiment, scarlet runner grown with corn in Wisconsin significantly improved dry-matter yield, almost doubling it over mono-cropped corn (Armstrong et al., 2008). The Romanian group concluded that runner beans were economical to intercrop with both corn and sunflowers and that the beans created an ideal microclimate for their intercrop (Hamburdă et al., 2015).
Because scarlet runners are more adapted to higher elevations in the tropics (much like coffee), it’s important to look for locally adapted varieties. In Central America and Mexico, there are landraces of scarlet runner that have been selected for many situations, including hot and dry climate areas (Delgado-Salinas, 1988). Some varieties are day-length dependent and may not set fruit the first year. So, if no varieties are specifically adapted to your area and you decide to select your own adaptation, patience is key.
Usually when beans are planted, the cotyledons rise out of the soil and open to catch light. Scarlet runner beans don’t do this at all. Their cotyledons stay beneath the soil surface, protected from harm. Another difference between scarlet runners and other beans is that they twine clockwise around poles, trellises, or other supports (Ashworth, 2002).
Scarlet runners are a bit of an anomaly with regard to the rest of the cover crops in this publication. The others are tried-and-true cover crops according to research, but scarlet runners have the potential to be cover crops according to indigenous use.
Stylosanthes (Stylosanthes spp.)
Stylosanthes is a many-branched perennial sub-shrub originating in South America. It has trifoliate leaves and yellow flowers. Unlike many legumes, its seeds come one to a pod, and they shatter readily, making mechanical harvesting difficult. It’s quite drought-tolerant once established, generally needing 700 to 900mm of rainfall per year, but highly susceptible to flooding (Stace and Edye, 1984). A general rule of thumb with Stylosanthes is that if swampy plants can live in your field, then Stylo cannot. Stylosanthes requires well drained soils that are not prone to crusting, due to its small seed size. It can tolerate acidic soils, but prefers neutral.
Stylosanthes has seen limited medicinal use. It’s mostly used for its anti-inflammatory properties for arthritis (Nageswaraiah et al., 2013), and swollen gums (Rekha et al., 2014).
There are a few species that are currently in use, including S. guianensis and S. hamata. There are an additional 40+ species of Stylosanthes, split between two sub-genera: Styposanthes and Stylosanthes (Maass and Sawkins, 2004). The genus is closely related to peanuts, which also evolved in the same region. The flowers of Stylosanthes and its ‘cousin’ Arachis look very similar, and they use the same inoculant. This publication concentrates on S. guianensis.
It’s imperative to know how cover crops can affect subsequent crops. If there is not available literature, try some test plots and verify compatibility.
Stylosanthes produces a limited amount of high quality dry matter per acre. Usually it yields less than five tons each year, but it can significantly raise the stocking rates of a given plot of land and can improve weight gain of livestock 60 to 160 pounds per head, per year. If not repeatedly defoliated by mowing or grazing, it can fail to persist intermixed with stands of tall grass. When repeatedly grazed, Stylosanthes forms a prostrate and creeping rosette; however, it’s best to mow or allow grazing while the plants are still in a succulent stage of growth. Once the plants become woody, mowing or overgrazing can kill them (Jingura et al., 2001). In addition to being an excellent feed for cattle, it is also a very effective feed for swine, which increases profits for producers by lowering the feed conversion ratio (Keoboualapheth and Mikled, 2003).
Stylo is being researched for its allelopahic properties. It has been shown to reduce weed biomass 80% at a rate of one ton of dry matter per acre. This led to a 40% increase in rice yield (Khanh et al., 2006), but another study showed that Stylosanthes negatively impacts cotton seed germination (Brown and Davis, 1992). It’s imperative to know how cover crops can affect subsequent crops. If there is not available literature, try some test plots and verify compatibility.
Mung bean, green gram (Vigna radiata)
Mung beans, also known as green gram, originated in Iran (Tomooka et al., 2003) or the Indian Sub-Continent (Lambrides and Godwin, 2006), depending on which reference you find first. It’s likely that it either spread quickly through trade or had multiple domestications, as suggested by Fuller (2007).
There are many, many cultivars and landraces of mung beans. The UDSA ARS GRIN database has over 4,600 accessions alone, not to mention the AVRDC accessions and the untold numbers of mung beans that have been cultivated by different families around the world (USDA ARS GRIN, 2016; AVDRC, 2016). Most representatives of this versatile crop are specifically bred for pulse production (dried legume seeds used for food). Mung beans are eaten in a variety of ways almost as vast as their many landraces, including sprouted. A few strains, though, have been recognized for another trait that is common in this Genus: prodigious vining. Mostly when we think of summer cover crops in the humid south, Iron-and-Clay Peas come to mind. These are Vigna unguicalata, a ‘cousin’ to the mung bean, which have been well discussed in the available literature and thus do not need to be covered here. One advantage that mung has over Iron-and-Clay Peas is its smaller seed size. This allows the seeds to germinate faster than Iron-and-Clay Peas, which is what makes them attractive to use as sprouts, but the practical agronomic purposes of faster sprouting times are less time in a vulnerable stage, less water needed to germinate, and better soil coverage to suppress weeds.
Maybe this faster germination explains why Butler et al. (2014) saw such a major difference between V. radiata and the other warm-season legumes they grew in sites from Oklahoma to Texas. It was by far the best performing on two of the three sites where it was grown and ranked second at the third site, behind Iron-and-Clay Peas by only 160 pounds per acre. The difference between V. radiata and its next-closest competitor at the other two sites was nearly 1,000 pounds per acre.
Mung beans can fix up to 250 pounds per acre of nitrogen when inoculated with the proper Rhizobium (Heuzé et al., 2015) from the cowpea group of inoculants.
There are a few more small-seeded Vigna species that are also of note in cover crop production, namely adzuki beans and rice beans, V. angularis and V. umbellata, respectively. They share many traits in common with mung beans.
References
Armstrong, K.L., K.A. Albrecht, J.G. Lauer, and H. Riday. 2008. Intercropping corn with Lablab bean, velvet bean and scarlet runner bean for forage. Crop Science. January-February.
Ashworth, S. 2002. Seed to Seed: Seed saving and growing techniques for vegetable gardeners. Seed Savers Exchange, Decorah, IA.
AVRDC. 2016. World Vegetable Center.
Bailey, G.E. 1915. Nitrating the Soil by Inoculated Legumes. University of Southern California Publications. Vol. 2, No. 1.
Bayer, C., L. Martin-Neto, J. Mielniczuk, C.N. Pillon, and L. Sangoi. 2000. Changes in soil organic matter fractions under subtropical no-till cropping systems. Soil Science Society of America Journal. Vol. 65, No. 5. p. 1473-1478.
Blanchart, E., C. Villenave, A. Viallatoux, B. Barthes, C. Girardin, A. Azontonde, and C. Feller. 2006. Long-term effect of a legume cover crop (Mucuna pruriens var. utilis) on the communities of soil macrofauna and nematofauna, under maize cultivation, in southern Benin. European Journal of Soil Biology. October. p. S136—S144.
Chitinase activity in Stylosanthes guianensis systematically protected against Colletotrichum goeosporoides.
Brown, A.E., and R.D. Davis. 1992. Journal of Phytopathology. Vol. 136. p. 247-256.
Bugg, R. L. 1991. Cover crops and control of arthropod pests of agriculture. In: W.L. Hargrove (ed.) Cover Crops for Clean Water. Proc of an Int Conf Soil and Water Conservation Society, SWCS, Ankeny, IA. p. 157-163.
Butler, T.J., S.M. Interrante, and J.L. Foster. 2014. Assessing the production and nutritive value of warm-season legumes in Oklahoma and Texas.Forage and Grazinglands. January. p. 1-6.
Caamal-Maldonado, J.A., J.J. Jimenez-Osornio, A. Torres-Barragan, and A.L. Anaya. 2001. The use of allelopathic legume cover and mulch species for weed control in cropping systems. Agronomy Journal. Vol. 93. p. 27—36.
Cairns, M. 2015. Shifting Cultivation and Environmental Change: Indigenous People Agriculture and Forest Conservation. Routledge, New York, NY.
Conway, M.J., K. McCosker, V. Osten, S. Coaker, and B.C. Pengelly. 2001. Butterfly Pea – A Legume Success Story in Cropping Lands of Central Queensland. In: B. Rowe, N. Mendham, and D. Donaghy, (eds.). Proceedings of the 10th Australian Agronomy Conference, Hobart. Tropical grasslands Journal. Vol. 39, No. 4. p. 124-127.
Creamer, N.G., and K.R. Baldwin. 2000. An Evaluation of Summer Cover Crops for Use in Vegetable Production Systems in North Carolina. HortScience. July. p. 600-603.
Dayal, D., A. Kumar, M.L. Swami, D. Machiwal, S. Manglassery, S.C. Vyas, and H. Kunpara. 2015. Management Practices for Improved Forage Production of Butterfly Pea (Clitoria ternatea). Chapter 18: p. 215-224. Devi Dayal, Deepesh Machiwal, Shamsudheen Mangalassery, and R.S. Tripathi (eds.) In: Improving Productivity of Drylands by Sustainable Resource Utilization & Management, Edition: 2016. New India Publishing Agency, New Delhi, India.
Debela, E., A. Tolera, L.O. Eik., and R. Salte. 2012. Condensed tannins from Sesbania sesban and Desmodium intortum as a means of Haemonchus contortus control in goats. Tropical Animal Health and Production. December. p. 1939-1944.
Delgado-Salinas, A. 1988. Variation, taxonomy, domestication, and germplasm potentialities in Phaseolus coccineus. p. 441—466. In: P. Gepts (ed.).Genetic Resources of Phaseolus Beans. Kluwer Academic, Dordrecht, Holland.
Duker-Eshun, G., J.W. Jaroszewski, W.A. Asomaning, F. Oppong-Boachie, and S.B. Christensen. 2004. Antiplasmodial constituents of Cajanus cajan. Phytotherapy Research. February. p. 128—130.
Ekpo, M., H. Mbagwu, C. Jackson, and M. Eno. 2011. Antimicrobial and wound healing activities of Centrosema pubescens (Leguminosae). Journal of Pharmacy and Clinical Sciences. April-June. p. 1-6.
FAO. No date a. Food and Agriculture Organization of the United Nations, Rome, Italy. Application of Nitrogen-Fixing Systems in Soil Management. FAO Soils Bulletin 49.
Grassland Species Profiles. No date b. Centrosema pubescens Benth.Food and Agriculture Organization of the United Nations.
FAO Ecocrop. 2007. Centrosema pubescens. Ecocrop.
Fox, J.E., J. Gulledge, E. Engelhaupt, M.E. Burow, and J.A. McLachlan. 2007. Pesticides reduce symbiotic efficiency of nitrogen-fixing rhizobia and host plants. Proceedings of the National Academy of Sciences. June 12. p. 10282—10287.
Fuller, D.Q. 2007. Contrasting patterns in crop domestication and domestication rates: recent archaeobotanical insights from the Old World. Annals of Botany. Vol. 100, No.5. p. 903—924.
Gage, D.J. 2004. Infection and invasion of roots by symbiotic, nitrogen-fixing rhizobia during nodulation of temperate legumes. Microbiology and Molecular Biology Reviews. June. p. 280-300.
Gillman, J. 2014. Warm-Season Native Grasses on Reclaimed Minelands–Landowner Management Guide. Land Management Fact Sheet. Missouri Geological Survey Division Publication 2082.
Gosper, H., and G. Murray. 2003. Covercrops for subtropical orchards. Agfact H6.3.10. NSW Agriculture’s Horticulture Program.
Hacker, J.B. 1992. Plant Resources of South-East Asia No. 4. Forages. Desmodium intortum (Miller) Urban. p. 114—115. In: L. ‘t Mannetje and R.M. Jones, (eds.). Pudoc Scientific Publishers, Wageningen, the Netherlands.
Hacker, J.B., C. Liu, L. Chungchu, and X. Minggang. 1997. Opportunities for selecting improved lines of Chamaecrista rotundifolia for southern China. In: J.M. Scott, D.A. MacLeod, Minggang Xu and A.J. Casanova, (eds.). Forages for the Red Soils Area of China. ACIAR Working Paper No. 55.
Hamburdă, S.B., N. Munteanu, V. Stoleru, G. Butnariu, G.C. Teliban, and L.D. Popa. 2014. Experimental results on runner bean cultivation (Phaseolus coccineus L.) in intercropping system. Lucrări Stiintifice Vol. 57, No. 1: Seria Horticultură
Hamburdă, S. B., N. Munteanu, and G.C. Teliban. 2015. Intercropping — a Successful System for Runner Bean (Phaseolus coccineus L.) Crop. Bulletin UASVM Horticulture. Vol. 72, No. 1. p. 81-86.
Hamel, P.B., and M. Chiltoskey. 1975. Cherokee plants and their uses: A 400 year history. Herald Publishing Company, Sylva, NC.
Hensley, David, Julie Yogi, and Joseph DeFrank. 1997. Perennial Peanut Groundcover. College of Tropical Agriculture and Human Resources, University of Hawaii at Manoa, Honolulu, HI.
Heuzé, V., and G. Tran. 2016. Centro (Centrosema molle). Feedipedia, a programme by INRA, CIRAD, AFZ and FAO.
Heuzé, V., G. Tran, D. Bastianelli, and F. Lebas. 2015. Mung bean (Vigna radiata). Feedipedia, a programme by INRA, CIRAD, AFZ and FAO.
Hue, N.V., G.R. Craddock, and F. Adams. 1985. Effect of Organic Acids on Aluminum Toxicity in Subsoils. SSSAJ. Vol. 50, No. 1. p. 28-34.
Hue, N.V., S. Vega, and J.A. Silva. 1999. Manganese Toxicity in a Hawaiian Oxisol Affected by Soil pH and Organic Amendments. SSSAJ. Vol. 65, No. 1. p. 153-160.
Ibrahim, K.A., E.A.E. ElSheikh, A.M. El Naim, and E.A. Mohammed. 2011. The effects of Bradyrhizobium inoculation on yield and yield components of hyacinth bean (Dolichos lablab L.). Australian Journal of Basic and Applied Sciences. Vol. 5, No. 6. p. 303-310.
Jeuffroy, M.H., C. Duthion, J.M. Meynard, and A. Pigeaire. 1990. Effect of a short period of high day temperatures during flowering on the seed number per pod of pea (Pisum sativum L). Agronomie, EDP Sciences. Vol. 10, No. 2. p. 139-145.
Jingura, R.M., S. Sibanda, and H. Hamudikuwanda. 2001. Tropical Grasslands. Vol. 35. p. 168-174.
Keoboualapheth, C., and C. Mikled. 2003. Growth performance of indigenous pigs fed with Stylosanthes guianensis CIAT 184 as replacement for rice bran. Livestock Research for Rural Development. Vol. 15, No. 9.
Khanh, T.D., N.H. Hong, D.Q. Nhan, S.L. Kim, I.M. Chung, and T.D. Xuan. 2006. Herbicidal activity of Stylosanthes guianensis and its phytotoxic components. Journal of Agronomy and Crop Science Vol. 192. p. 427-433.
Kirschbaum, M.U.F. 1995. The temperature dependence of soil organic matter decomposition, and the effect of global warming on soil organic C storage. Soil Biology and Biochemistry. June. p. 753—760.
Lambrides, C.J., and I.D. Godwin. 2006. Genome Mapping and Molecular Breeding in Plants, 3. Mungbean. p. 69-90. In: K. Chittarajan. Springer, New York, NY.
Lampariello, L.R., A. Cortelazzo, R. Guerranti, C. Sticozzi, and G. Valacchi. 2012. The magic velvet bean of Mucuna pruriens.The Journal of Traditional and Complimentary Medicine. Vol. 2, No. 4. p. 331-339.
Maass, B., and M. Sawkins. 2004. History, relationships and diversity among Stylosanthes species of commercial significance. In: Chakraborty S. High-Yielding Anthracnose-Resistant Stylosanthes for Agricultural Systems (PDF). Australian Centre for International Agricultural Research (ACIAR). p. 9—26.
Mahdi, A.A., and I.M.A. Atabani, 1992. Response of Braydrhizobium inoculated soybean and lablab bean to inoculation with vesicular arbuscular mycorrhizae. Experimental Agriculture. Vol. 28. p. 243-249.
Merel, H., A. Akoègninou, and J. van der Maesen. 2004. Medicinal plants used to treat malaria in Southern Benin. Economic Botany. December. p. S239-S252.
Miavitz, E., and R. Rouse. 2002. Rhizomal perennial peanut in the urban landscape. Proceedings of the Florida State Horticultural Society. Vol. 115. p. 136-138.
Midega, C.A.O., D. Salifu, T.J. Bruce, J. Pittchar, J.A. Pickett, and Z.R. Khan. 2014.Cumulative effects and economic benefits of intercropping maize with food legumes on Striga hermonthica infestation. Field Crops Research. Vol. 155. p. 144-152.
Mukherjee, P.K., V. Kumar, N.S. Kumar, and M. Heinrich. 2008. The Ayurvedic medicine Clitoria ternatea—from traditional use to scientific assessment. Journal of Ethnopharmacology. Vol. 120. p. 291—301.
Muir, J.P. 2012. Perspectives on forage legume systems for the tallgrass and mixed-grass prairies of the Southern Great Plains of Texas and Oklahoma. Crop Science. Vol. 53. September-October. p. 1971-1979.
Muir, J.P., T.J. Butler, W.R. Ocumpaugh, and C.E. Simpson. 2010. ‘Latitude 34’, a perennial peanut for cool, dry climates. Journal of Plant Registrations. Vol. 4. p. 106—108.
Nageswaraiah, B., N. Dorababu, G.R. Battu, S. Satyanarayana, Y. Nagendra, and B. Mohan. 2013. Anti-inflammatory activity of methanolic extract of Stylosanthes fruticosa (Retz.) leaves. International Journal of Chemical and Pharmaceutical Sciences. September. Vol. 4, No. 3. p. 123-126.
Oblisami, G. 1974. Studies on the Rhizobium and nodulation pattern in a forage legume, Clitoria ternatea. Proceedings of the National Academy of Sciences, India Section B: Biological Sciences. Vol. 40. p. 618-623.
Odeny, D.A. 2007. The potential of pigeonpea (Cajanus cajan (L.) Millsp.) in Africa. Natural Resources Forum. Vol. 31. p. 297—305.
O’Gara, F. 2005. Evaluation of Wynn Cassia (Chamaecrista rotundifolia) as a pasture and hay crop for the Douglas Daly area of the Northern Territory. Technical Bulletin No. 316. June.
Okpara, D.A., J.E.G. Ikeorgu, and J.C. Njoku. 2005. Potential of cover crops for short fallow replacement in low-input systems of maize production in the humid Tropics. Tropical and Subtropical Agroecosystems. Vol. 5. p. 109-116.
Omokanye, A.T. 2001. Seed production, herbage residue and crude protein content of Centro (Centrosema pubescens) in the year of establishment at Shika, Nigeria Tropicultura. Vol. 19, No. 4. p. 176-179.
Palm, C.A. 1995. Contributions of agroforestry trees to nutrient requirements of intercropped plants. Agroforestry Systems. Vol. 30. p. 105-124.
Pickett J., C.M. Woodcock, C.A.O. Midega, and Z.R. Khan. 2014. “Push-pull farming systems.” Current Opinion in Biotechnology. Vol. 26C. p. 125-132.
Pillai. U.P., and D. McGarry. 1999. Structure Repair of a Compacted Vertisol with Wet-Dry Cycles and Crops. Soil Science Society of America Journal. December. p. 201-210.
Rekha, D., A. Panneerselvam, and N. Thajuddin. 2014. Studies on medicinal plants of A.V.V.M. Sri Pushpam College Campus Thanjavur district of Tamil nadu, Southern India. World Journal of Pharmaceutical Research. Vol. 3, No. 5. p. 785-820.
Reid, R., and D.F. Sinclair. 1980. An Evaluation of a Collection of Clitoria ternatea for Forage and Grain Production. Genetic Resources Communication. Vol. 1. p. 1-8.
Rouse, R.E., R.M. Muchovej, and J.J. Mullahey. 2001. Guide to using perennial peanut as a cover crop in citrus. Florida Cooperative Extension Service Factsheet HS-805.
Salako, F.K., and G. Tian. 2003. Soil water depletion under various leguminous cover crops in the derived savanna of West Africa. Agriculture, Ecosystems and Environment. Vol. 100. p. 173-180.
Sheahan, C.M. 2012. Plant Guide for Lablab (Lablab purpureus). USDA-Natural Resources Conservation Service, Cape May Plant Materials Center. Cape May, NJ.
Sogbedji, J., H.M. van Es, and K.L. Agbeko. 2006. Cover cropping and nutrient management strategies for maize production in Western Africa. Agronomy Journal. Vol. 98, No.4. June. p. 883-889.
Solomon, T. and M. Flores. 1994. Intercropping corn and frijol chinapopo (Phaseolus coccineus). CIDDICO Tegucigalpa MDC, Honduras.
Soti, P.G., S. Rugg, and A. Racelis. 2016. Potential of cover crops in promoting mycorrhizaldiversity and soil quality in organic farms. Journal of Agricultural Science. Vol. 8, No. 8. p. 42-47.
Stace, H.M., and L.A. Edye . (eds.). 1984. The biology and agronomy of Stylosanthes. Academic Press, Sydney, Australia.
Staples, I.P. 1992.Clitoria ternatea L. In: L. ‘t Mannetje and R.M. Jones (eds.). Plant Resources of South-East Asia No. 4. Forages. Pudoc Scientific Publishers, Wageningen, the Netherlands. p. 94-96.
Tanimu, J., E.N.O. Iwuafor, A.C. Odunze, and G. Tian. 2007. Effect of incorporation of leguminous cover crops on yield and yield components of maize. World Journal of Agricultural Sciences. Vol. 3, No. 2. p. 243-249.
Tian, G., G.O. Kolawole, B.T. Kang, and G. Kirchof. 2000. Nitrogen fertilizer replacement indexes of legume cover crops in the derived savanna of West Africa. Plant and Soil. Vol. 224. p. 287-296.
Tomooka, N., D.A. Vaughan, H. Moss, N. Mixted. 2003.The Asian Vigna: genus Vigna subgenus Ceratotropis genetic resources. Kluwer, New York, New York.
USDA ARS GRIN. 2016. National Plant Germplasm System. U.S.
USDA-NRCS. 2016. Introduced, Invasive, and Noxious Plants.
Valenzuela, H. 2011. Hanai’Ai /The Food Provider. Pigeon peas: a multipurpose crop for Hawaii. March-April-May. p. 1-8.
Valenzuela, H., and J. Smith. 2002. Lablab. Sustainable Agriculture Green Manure Crops. SA GM-7. CTAHR, Manoa, Hawaii.
Vieira, F.C.B., C. Bayer, J.A. Zanatta, J. Mielniczuk, and J. Six. 2009. Building up organic matter in a subtropical aleudult under legume Cover-Crop-Based Rotations. Soil Science Society of America Journal. September—October. p. 1699-1706.
Wang, K-H. 2012. Cover crops as insectary plants to enhance above and below ground beneficial organisms. Hanai’Ai/The Food Provider. March-April-May. p. 1-7.
White, R.H., A.D. Worsham, and U. Blum. 1989. Allelopathic potential of legume debris and aqueous extracts. Weed Science. September 1989. p. 674-679.
Yusof, N.A.B.A. 2015. Investigation on natural blue colorant of Clitoria ternatea Fabacaeae (bunga telang): The effects of stabilizers to extracts. Chemical & Natural Resources Engineering, University Malaysia, Pahang.
Further Resources
Videos
Cover Crop Options for Hot and Humid Areas
Mimicking Nature: One Farmer’s Cover Crop History
Cover Crop Management for the Southeast
Online Resources
Cover Crops and Green Manures for Hawai’i
Sustainable and Organic Agriculture Program, University of Hawai’i College of Tropical Agriculture and Human Resources
Cover Crops for the Southeast
USDA-NRCS Georgia Plant Materials Program
Appendix 1: Inoculant Groups
i. Alfalfa group
Common name Scientific name
Alfalfa Medicago sativa
Button-clover M. orbicularis
California bur-clover M. denticulata
Spotted bur-clover M. arabica
Black medic M. lupulina
Snail bur-clover M. scutellata
Tubercle bur-clover M. tuberculata
Little bur-clover M. minima
Tifton bur-clover M. rigidula
Yellow alfalfa M. falcata
White sweet clover Melilotus alba
Huban sweet clover M. alba annua
Yellow sweet clover M. officinalis
Bitter clover (sour clover) M. indica
Fenugreek Trigonella foenumgraceum
ii. Clover group
Alsike clover Trifolium hybridum
Crimson clover T. incarnatum
Hop clover T. agrarium
Small hop clover T. dubium
Large hop clover T. procumbens
Rabbit foot clover T. arvense
Red clover T. pratense
White clover T. repens
Ladino clover T. repens (giganteum)
Subclover T. subterraneum
Strawberry clover T. fragiferum
Berseem clover T. alexandrinum
Cluster clover T. glomeratum
Zigzag clover T. medium
Ball clover T. nigrescens
Persian clover T. resupinatum
Carolina clover T. carolinianum
Rose clover T. hirtum
Buffalo clover T. reflexum
Hungarian clover T. pannonicum
Seaside clover T. wormskjoldii
Lappa clover T. lappaceum
Bigflower clover T. michelianum
Puff clover T. fucatum
iii. Pea and vetch group
Field pea Pisum arvercse
Garden pea P. sativum
Australian winter pea P. sativum (var arvense)
Common vetch Vicia sativa
Hairy or winter vetch V. villosa
Horse or broad bean V. faba
Narrow leaf vetch V. angustiffolia
Purple vetch V. atropurpurea
Monantha vetch V. articulata
Sweet pea Lathyrus odoratus
Rough pea L. hirsutus
Tangier pea L. tingitanus
Flat pea L. sylvestris
Lentil Lens culinaris (esculenta)
iv. Cowpea group
Cowpea Vigna sinensis
Asparagus bean V. sesquipedalis
Common lespedeza Lespedeza striata
Korean lespedeza L. stipulacea
Sericea lespedeza L. cuneata
Slender bush clover L. virginica
Striped crotalaria Crotalaria mucronata
Sun crotalaria (Sunnhemp) C. juncea
Winged crotalaria C. sagittalis
Florida beggarweed Desmodium tortuosum
Tick trefoil D. illinoense
Hoary tickclover D. canescens
Kudzu Pueraria thunbergiana
Alyce clover Alysicarpus vaginalis
Erythrina indica
Pigeon pea Cajanus cajan (indicus)
Guar (cluster bean) Cyamopsis tetragonoloba
Jackbean (horse bean) Canavalia ensiformis
Groundnut (peanut) Arachis hypogaea
Velvet bean Mucuna deeringianum
Adzuki bean P. angularis
Mat bean P. aconitifolius
Mung bean P. aureus
Tepary bean P. acutifolius var latifolius
Acacia Acacia linifolia
Kangaroo-horn A. armata
Wild indigo Baptisia tinctoria
Hairy indigo Indigofera hirsuta
Partridge-pea Chamaecrista fasciculata
v. Bean group
Garden bean, kidney bean navy bean, pinto bean Phaseolus vulgaris
Lima bean Phaseolus lunatus (macrocarpus)
Scarlet runner bean P. coccineus (multiflorus)
vi. Lupine group
Blue lupine Lupinus angustifolius
Yellow lupine L. luteus
White lupine L. albus
Washington lupine L. polyphyllus
Sundial L. perennis
Texas bluebonnet L. subcarnosus
Serradella Ornithopus sativus
vii. Soybean group
All varieties of soybean Glycine max (Soja max)
viii. Unique strains
Common name Scientific name
Birdsfoot trefoil Lotus corniculatus
Big trefoil L. uliginosus
Foxtail dalea Dalea alopecuroides
Black locust Robinia pseudoacacia
Trailing wild bean Strophostyles helvola
Hemp sesbania Sesbania exaltata
Kura clover Trifolium ambiguum
Sanfoin Onobrychis vulgaris (sativus)
Crown vetch Coronilla varia
Siberian pea shrub Caragana arborescens
Garbanzo (chick pea) Cicer arietinum
Lead plant Amorpha canescens
French Honeysuckle Hedysarum coronarium
Adapted from Application of Nitrogen-Fixing Systems in Soil Management (FAO, no date.)
Cover Crop Options for Hot and Humid Areas
By Justin Duncan, NCAT Agriculture Specialist
Published April 2017
©NCAT
IP535
This publication is produced by the National Center for Appropriate Technology through the ATTRA Sustainable Agriculture program, under a cooperative agreement with USDA Rural Development. This publication was also made possible in part by funding from the National Institute of Food and Agriculture, U.S. Department of Agriculture, award number 2013-51106-20970. ATTRA.NCAT.ORG.
Related Resources
Publications:
- Overview of Cover Crops and Green Manures
- No-Till Case Study, Miller Farm: Restoring Grazing Land with Cover Crops
- No-Till Case Study, Richter Farm: Cover Crop Cocktails in a Forage-Based System
- Pigeon Pea (Cajanus cajun): A Cover Crop for Hot and Humid Areas
- Partridge Pea: A Cover Crop for Hot and Humid Areas
- Scarlet Runner Bean: A Cover Crop for Hot and Humid Areas
- Butterfly Pea: A Cover Crop for Hot and Humid Areas
- Velvet Bean (Mucuna pruriens var utilis): A Cover Crop for Hot and Humid Areas
Videos: